Gambogic acid induces mitochondria-dependent apoptosis by modulation of Bcl-2 and Bax in mantle cell lymphoma JeKo-1 cells
Introduction
Mantle Cell Lymphoma (MCL) is an incurable B-cell non-Hodgkin Lymphoma despite current available treatments including radiation, chemotherapy or hematopoietic stem cell transplantation. The median survival of the majority of patients is in the range of 3 to 5 years (1). Therefore, novel, less toxic and more effective anti-carcinogens are needed to improve the curative effect on MCL.
Garcinia hanburyi is a traditional herbal medicine used in anti-inflammation and hemostasis in South Asia. Gambogic acid (GA) is the main active component extracted from it. There is accumulating evidence that GA has antitumor effect both in vitro and in vivo (2). It exhibits remarkable inhibition of proliferation, induction of apoptosis, reversion of multidrug resistance and antiangiogenesis. Although the underlying mechanism is not fully understood, studies have demonstrated that GA induces cytotoxity in variety of tumor cells by cell cycle arresting, interaction with oncogene c-MYC and inhibition of the telomerase activity (3). Moreover, GA has shown to down-regulate the expression of SRC-3 and then inhibit Akt pathway in leukemia K562 cells (4). GA also induces mitochondrial outer membrane permeabilization via P53 and Bcl-2 family in human breast MCF-7 cancer cells (5). In addition, GA can inhibit NF-κB signaling pathway and potentiate apoptosis through its interaction with the transferin receptor (6). Recently, it is reported that GA induces reactive oxygen species (ROS) accumulation and triggers the mitochondrial signaling pathway in human hepatoma SMMC-7721 cells (7).
Although many reports have stated that GA promotes and regulates tumor cell apoptosis, whether GA can mediate antitumor effect on MCL cells has not been reported until now. In this study, we investigated the apoptosis effects of GA on MCL cells and the underlying molecular mechanism. Our results demonstrated that GA inhibited the proliferation of human MCL JeKo-1 cells and induced apoptosis by reducing the membrane potential of mitochondria, activating caspases-3, -8 and -9 and decreasing the ratio of Bcl-2 and Bax.
Materials and methods
Reagents
GA (Sigma Chemical Company, USA) was dissolved in DMSO (Sigma Chemical Company, USA) as a stock solution and kept at –20 °C. The solution was diluted with RPMI-1640 medium (Gibco Company, USA) to various working concentrations when used.
Cell culture
The MCL JeKo-1 cell line was a kind gift from Dr. Mi Jianqing (Shanghai Institute of Hematology, Shanghai Jiao Tong University, China). Normal bone marrow mononuclear cells from hematopoietic stem cell transplant donors were isolated by density gradient centrifugation using Ficoll-Hypaque Solution (Tianjin Hanyang Biologicals Technology Co. Ltd., China). Then the cells were resuspended in culture medium consisting of RPMI 1640 at a final density of 2×106 cells/mL. The two types of cells above were grown in RPMI-1640 medium supplemented with 10% heat-inactivated bovine serum (Hyclone Company, USA), 100 units/mL penicillin G and 100 μg/mL streptomycin under a humidified atmosphere of 5% CO2 at 37 °C.
Cell proliferation assay
A total of 1×104 JeKo-1 cells per well were plated in 96-well plates and cultured for 24 hrs in normal conditions. At the indicated time (12, 24, and 36 hrs) points after various concentrations of GA treatment (0.0625-4 μg/mL), 10 µL of Cell Counting Kit 8 (CCK-8) (Dojindo Company, Japan) reagent were added to each well and incubated at 37 °C for 3 h. Absorbance was measured at 450 nm in a spectrophotometer (CRAIC Technologies, UV-2802PCU, SA). Each experiment was performed in triplicate and repeated at least three times.
Cell-cycle analysis
JeKo-1 cells were incubated with GA (0, 0.5, 0.1 and 2 μg/mL, respectively) for 24 hrs, collected and washed with PBS buffer, fixed in 75% alcohol overnight at –20 °C. After centrifugation, 1 mL PI (Tianjin Hanyang Biologicals Technology Co. Ltd., China) was added and incubated for 15 min in the dark. The samples were analyzed with BD FACScan flow cytometry (BD Biosciences, CA) using Cell Quest Software (BD Biosciences, CA).
Apoptosis assay
JeKo-1 Cells in early and late stages of apoptosis were detected using an Annexin V-FITC Apoptosis Detection kit (Tianjin Hanyang Biologicals Technology Co. Ltd., China). (1-5)×105 cells per well were exposed to various concentrations of GA (0, 0.5, 1 and 2 μg/mL) and incubated for 24 hrs prior to analysis. Cells were harvested and washed with PBS, then resuspended in 500 μL of Annexin V binding buffer, followed by addition of 5 μL of Annexin V-FITC and 5 μL PI. The samples were incubated in the dark for 15 min at room temperature and the analysis of apoptotic cells was performed using BD Cell Quest Software.
Mitochondrial transmembrane potential (Ψm) assay
After culture with various concentrations of GA (0, 0.5, 1.0, 2.0 μg/mL) for 24 hrs, the JeKo-1 cells were washed with PBS 3 times, 1 µM 5,5',6,6' -tetrachloro-1,1',3,3' -tetraethyl-benzimidazolyl-carbocyanine iodide (JC-1; Molecular Probes, USA) was added to the cells for 20 min. After the JC-1 was removed, the cells were washed with PBS and resuspended in PBS. The amount of JC-1 retained by 10,000 cells per sample was measured at 488 and 575 nm with a flow cytometry and analyzed with Cell Quest Alias software. In non-apoptotic cells, JC-1 enters the negatively-charged mitochondria where it aggregates and turns red. However, in cells undergoing apoptosis, where Ψm has collapsed, JC-1 exists as monomers in the cytosol and turns green.
Detection of caspase activity
The activity of caspases -3, -8 and -9 was measured using caspases colorimetric assay kits (Kaiji Company, China). After culture with various concentrations of GA (0, 0.5, 1 and 2 μg/mL) for 24 hrs, cells were washed with PBS and lysed on ice in 50 μL of cold lysis buffer for 20 min. Cell lysates were centrifuged at 10,000 g for 1 min to precipitate cellular debris. The protein concentration of each sample was measured. 50 μL protein extracts were incubated with 50 μL reaction buffer and 5 μL caspase-3 or -8 or -9 substrate, in 37 °C for 4 hours and assays were performed in triplicate in 96-well plates by a spectrophotometer at 405 nm. Caspase activity was calculated by comparison of the absorbance from apoptotic samples with that of the control.
Western blotting
After culture with various concentrations of GA (0, 0.5, 1 and 2 μg/mL) for 24 h, the cells were harvested in cell lysis solution (BioDev-Tech Company, China), then protein was extracted. 30 µg proteins were separated by 12% SDS- PAGE and electro-transferred onto nitrocellulose membranes. After blocking with 5% nonfat-dried milk in PBS for 1 hour at room temperature, the membranes were incubated with 1:5,000 Bcl-2 and Bax specific monoclonal antibody (Abcam Company, USA) for 2 h at room temperature, respectively. After incubation with 1:5,000 horseradish peroxidase-conjugated secondary antibody (Beijing Zhong Shan-Golden Bridge Biological Technology Company, China) for 1 h at room temperature, the immunoblotting signals were visualized using a Western Luminescent Detection kit (Vigorous Biotechnology, China). The results were quantified by densitometry and the density of immunoblotting was analyzed by scanning X-ray film with Quantitative One Software.
Immunocytochemical detection of ki-67 proteins
The samples with or without GA treated JeKo-1 cells were initially fixed in 1.5% agarose incubated for 15 min at room temperature. Three-micrometer sections from the paraffin block were placed on adhesive-coated slides. In a heated antigen retrieval process, the slides were placed in EDTA buffer (pH 8.0) and heated for 2 min in the steamer. The slides were incubated overnight at 4 °C with monoclonal antibodies to Ki-67 with 1:50 dilution in bovine serum albumin before incubating with second antibody at room temperature for 20 min. Color was developed by reaction with DAB solution for 10 min followed by counterstaining with Harris hematoxylin, dehydrated, coverslipped, and reviewed under light microscope. Ki-67 expression was exclusively nuclear.
Statistical analysis
Data were expressed as the mean ± SD unless otherwise noted. The differences between groups were analyzed using a two-tailed Student’s t-test and the null hypothesis was rejected at the 0.05 level.
Results
GA inhibits JeKo-1 cell growth in vitro
To analyze the effect of GA on cell growth, we used the CCK-8 assay to measure JeKo-1 cell viability after being exposed to various concentrations of GA for 12, 24, 36 hrs. Figure 1 shows that GA significantly inhibited the growth of JeKo-1 cells in a dose- and time-dependent manner. The maximum GA-induced cytotoxity was evident at 36 hrs exposure to 4 μg/mL GA. The IC50 of 12, 24 and 36 hrs of GA treatment for JeKo-1 cells was 0.73, 0.72 and 0.55 μg/mL respectively.
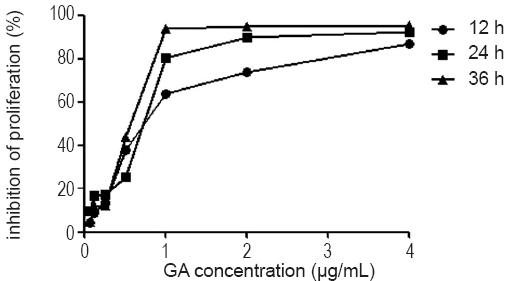
As we known, Ki-67 protein is an excellent and positive marker to determine the growth fraction of a given cell population (8). So we used Ki-67 to further verify that GA inhibited JeKo-1 cells growth. Figure 2 demonstrated that the expression of ki-67 decreased dramatically after incubation with various concentration of GA for 24 hrs (from 60.90% in control group to 8.80% in treated group with GA 2 μg/mL).
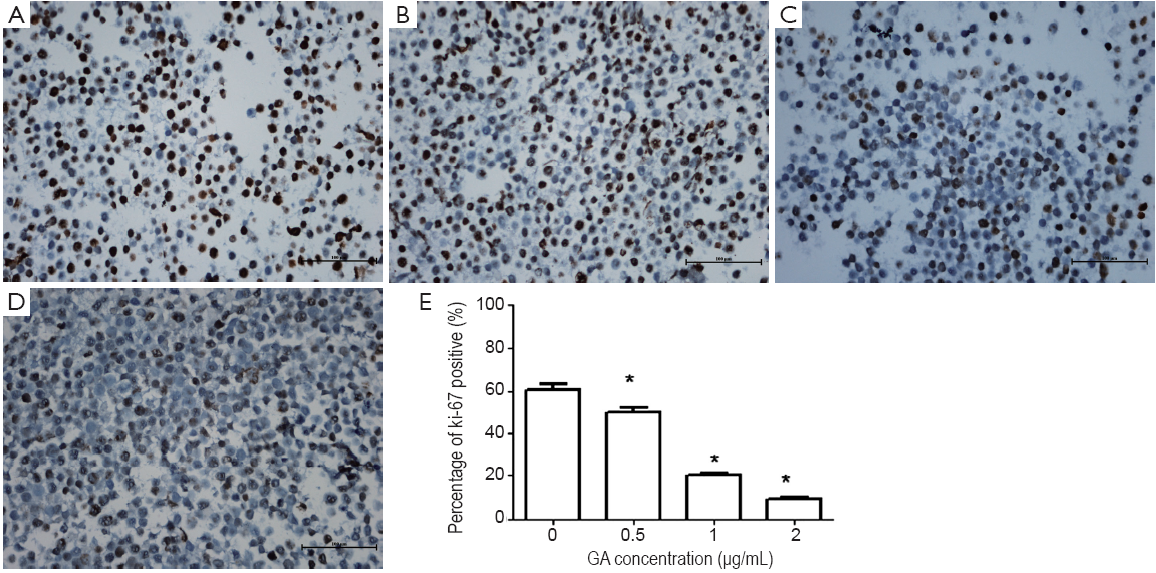
GA induces apoptosis in JeKo-1 cells without cell cycle arresting but not in normal bone marrow cells
Inhibition of cell proliferation could result from the induction of apoptosis and/or cell growth arrest. We first observed apoptosis rate using flow cytometry analysis in JeKo-1 cells affected by GA. Figure 3 showed that GA increased the percentage of JeKo-1cells apoptosis in a dose-dependent manner (from 8.3% to 48.7%) (P<0.05). However, as shown in Figure 4, the same concentration gradient of GA did not affect apoptosis in normal bone marrow cells at low concentration (<2.0 μg/mL) (P>0.05). Next, we measured the cell cycle distribution in JeKo-1 cells cultured by GA and found that GA did not affect it at all (Figure 5).
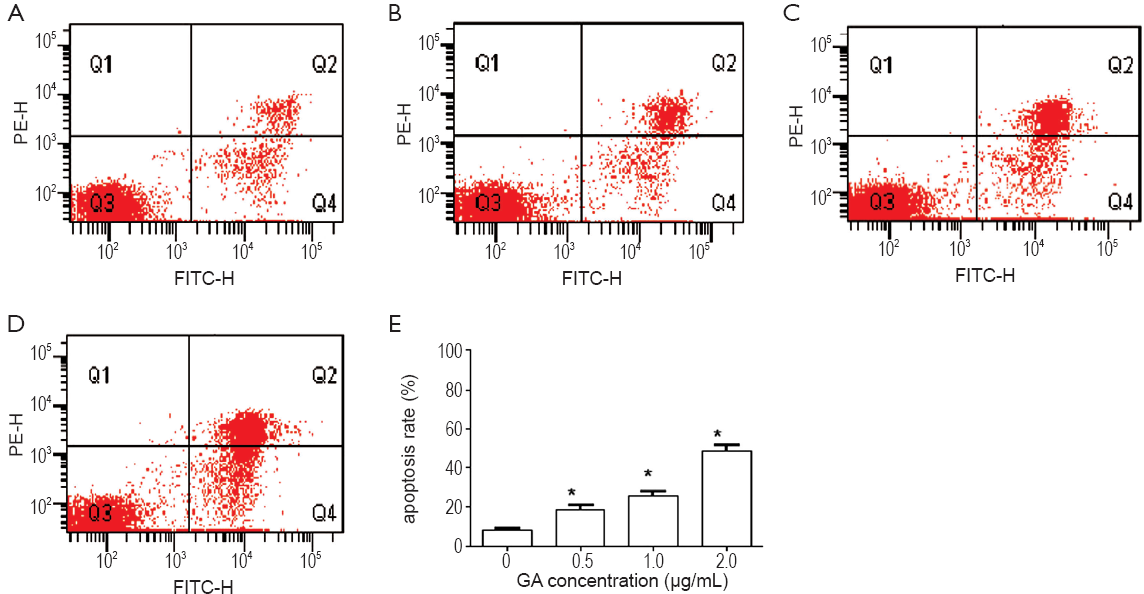
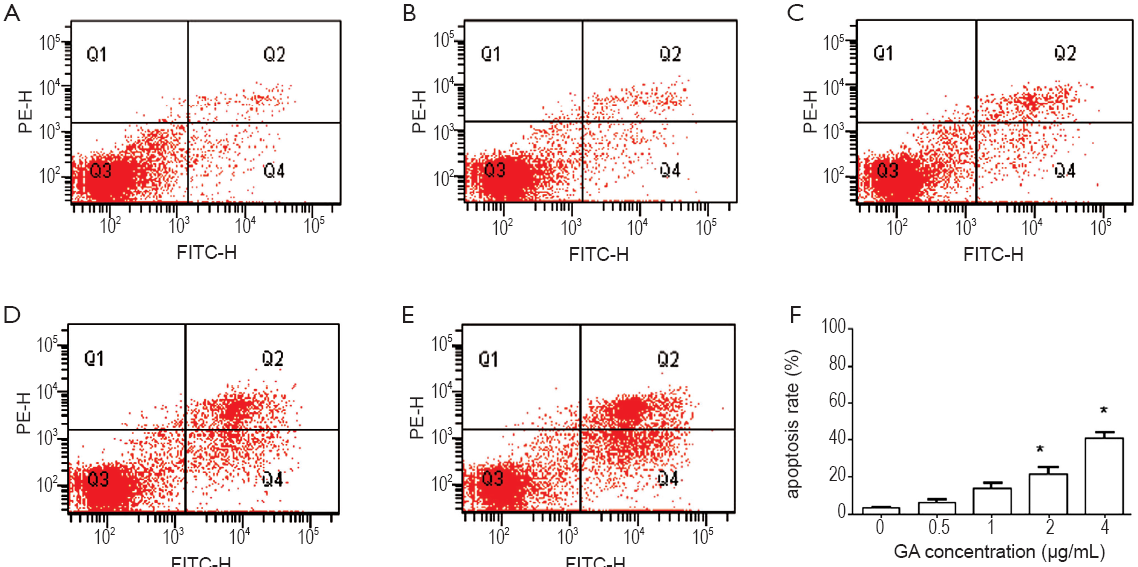
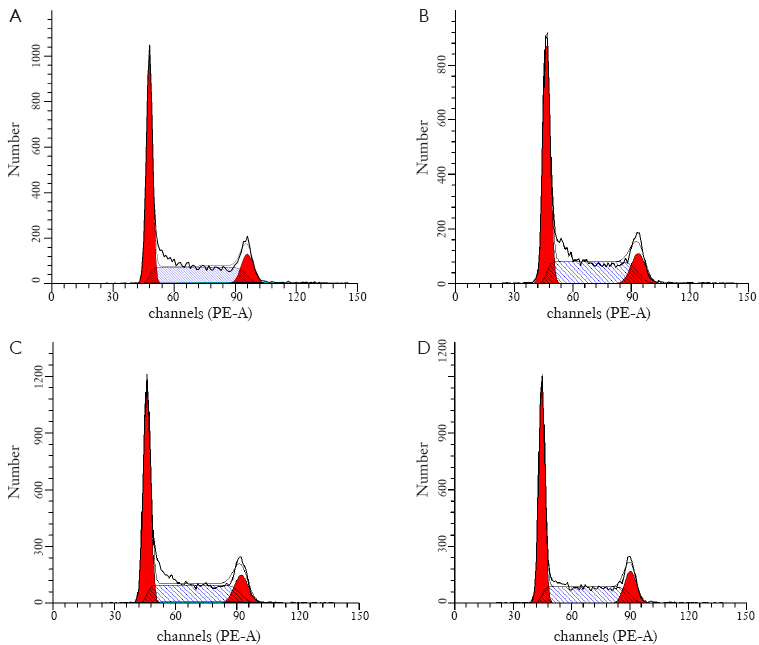
GA promoted the activities of caspase-3, -8 and -9 in JeKo-1 cells
Since caspase-3 is a common downstream apoptosis effector, which is processed and activated by caspase-8 or -9 and digested into the heterodimeric from 12-17 kDa by mitochondrial pathways or through the activation of death-domain containing receptors (9), we investigated the activation of caspases-3, -8 and -9 in GA-induced JeKo-1 cells. The results showed that GA activated caspases-3, -8 and -9 in a dose-dependent manner (Figure 6).
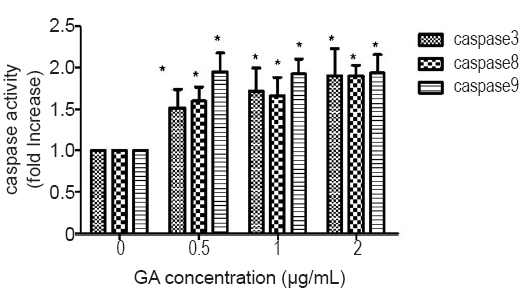
GA changes the membrane potential of mitochondria in JeKo-1cells
In addition, Loss of Ψm is a crucial step in the apoptotic process and is lethal to cells because it leads to the release of diverse pro-apoptotic factors from the mitochondria into the cytoplasm (10). In this study, we used the unique cationic dye JC-1 to analyze the membrane potential of the mitochondria in JeKo-1 cells after GA treatment. Our data showed there is a significant depletion of Ψm in JeKo-1 cells treated with 1.0 and 2.0 ng/mL GA for 24 hours compared with the control (Figure 7).
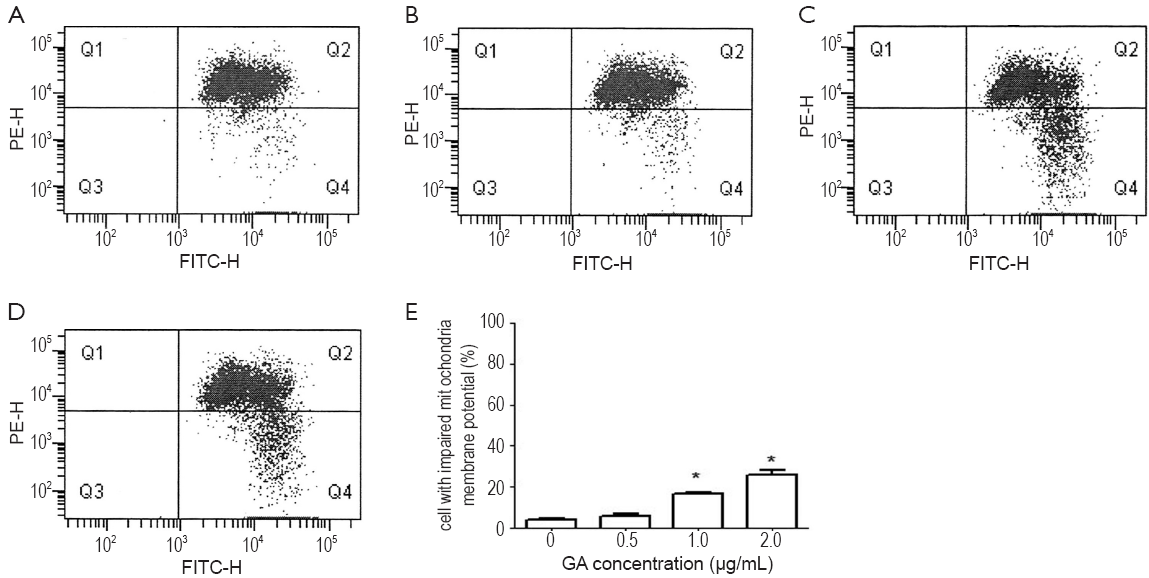
GA decreased the ratio of BCL-2 and Bax
The Bcl-2 family of proteins includes pro- and anti-apoptotic factors acting at mitochondrial membranes. The Bcl-2 family of proteins are key regulators of mitochondrial permeability (11). Therefore, we investigated whether the mitochondria-mediated apoptosis in JeKo-1 cells induced by GA was modulated by Bcl-2 and Bax protein. Western blotting revealed that there was a significant decrease in Bcl-2 expression and increase in Bax expression in a dose-dependent manner after the GA treatment. The ratio of Bcl-2/Bax was reduced (Figure 8).
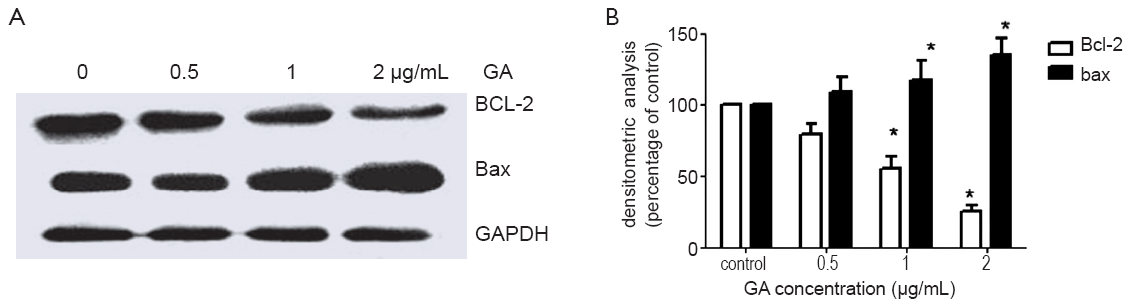
Discussion
Accumulating evidence has shown that GA has shown anti-tumor effects against a variety of human cancers cell lines inluding hepatoma, breast cancer, gastric carcinoma and lung carcinoma (2,12-15). To our knowledge, this is the first report by now to suggest that GA could inhibit the proliferation and induce apoptosis in human JeKo-1 MCL cell lines. Moreover, we found that after incubating with 2 μg/mL GA for 24 hrs, only less than 21.8±2.8% normal bone marrow cells appeared apoptosis while 48.7±2.6% JeKo-1 cells did. Guo et al. also reported that GA had no dramatical effect on the count of peripheral leukocyte and marrow karyote (12). Using 125I-labled GA, they found that GA had higher binding activity with human hepatoma SMMC-7721 cells than human normal embryon hepatic L02 cells and GA had longer retention time in tumor than that in liver, lung and other organs. These results partly suggested that GA might be a potential anticancer candidate for clinical applications.
In many report, GA induced apoptosis accompany with cell cycle arrest (12,16,17). However, our study showed that GA did not interfere with cell cycle in JeKo-1 cells. Apoptosis can be triggered by multiple pathways and growth arresting may be the independent events during apoptosis (18). Another study also reported that GA can significantly inhibit the proliferation of K562 cells without influence on cell cycles (19).
Mitochondria play a crucial role in determining whether to die during the common apoptotic signaling pathway. The dissipation of the inner mitochondrial transmembrane potential marks the point of no return during the apoptotic program and occurs prior to DNA fragmentation (20). Thus, the evaluation of mitochondrial transmembrane potential depolarization is of critical importance for the assessment of apoptosis (21,22). Our results showed that GA reduced the level of mitochondrial transmembrane potential suggesting that mitochondria involves in the GA-induced apoptosis in JeKo-1 cells.
As we known, the Bcl-2 family is made up of outer mitochondrial membrane that can regulate cell apoptosis. As an anti-apoptosis Bcl-2 protein, Bcl-2 can maintain mitochondrial homeostasis and prevent mitochondrial release of cytochrome c while Bax, as a pro-apoptosis Bcl-2 protein, can down-regulate mitochondrial permeability and promote release of cytochrome c (23). Therefore, the relative ratio of Bcl-2/Bax of the cells seems to determine whether or not apoptosis occurs. Our results indicated that the relative ratio of Bcl-2/Bax was significantly decreased. Specifically, there was a significant decrease in Bcl-2 (P<0.05) and slightly increase in Bax (P>0.05). Further study is needed to know whether other BCL-2 family members also interact and involve in regulation of apoptosis in GA-induced JeKo-1 cells.
Apoptosis caused by mitochondria involves in activation of caspases. In the intrinsic way, caspase-9 is activated by downstream of mitochondrial permeability transition (ψm), which is mediated by cytochrome c release and the decreasing ratio between Bcl-2 and Bax (24). Caspases-9 are initiator caspase that in turn activate downstream executioner caspases, such as caspases-3, -6 and -7. In the extrinsic way, Fas-associated death domain protein activates the caspase-8 which in sequence activates downstream executioner caspase-3 or -7. As our results shown, there is a significant increase of active caspase-3, -8 and -9 in GA-treated JeKo-1 cells, which means GA might induce apoptosis through both intrinsic and extrinsic ways.
In conclusion, GA induced apoptosis in human MCL JeKo-1 cells by regulating Bcl-2/Bax and activating caspase-3, -8 and -9 via mitochondrial pathway without affecting cell cycle. Moreover, under the same condition of inducing apoptosis in JeKo-1 cells, GA showed little effect on apoptosis of normal bone marrow cells. Exploring more effect and mechanism of GA in cancer cells will provide more information for its possible application in cancer therapy.
Acknowledgements
This work was supported by a grant from the Key Project supported by medical science and technology development Foundation of Nanjing Department of Health (No. ZKX09016).
Disclosure: The authors declare no conflict of interest.
References
- Bautista-Quach MA, Ake CD, Chen M, et al. Gastrointestinal lymphomas: Morphology, immunophenotype and molecular features. J Gastrointest Oncol 2012;3:209-225.
- Wu ZQ, Guo QL, You QD, et al. Gambogic acid inhibits proliferation of human lung carcinoma SPC-A1 cells in vivo and in vitro and represses telomerase activity and telomerase reverse transcriptase mRNA expression in the cells. Biol Pharm Bull 2004;27:1769-74.
- Guo QL, Lin SS, You QD, et al. Inhibition of human telomerase reverse transcriptase gene expression by gambogic acid in human hepatoma SMMC-7721 cells. Life Sci 2006;78:1238-45.
- Colo GP, Rubio MF, Nojek IM, et al. The p160 nuclear receptor co-activator RAC3 exerts an anti-apoptotic role through a cytoplasmatic action. Oncogene 2008;27:2430-44.
- Gu H, Rao S, Zhao J, et al. Gambogic acid reduced bcl-2 expression via p53 in human breast MCF-7 cancer cells. J Cancer Res Clin Oncol 2009;135:1777-82.
- Pandey MK, Sung B, Ahn KS, et al. Gambogic acid, a novel ligand for transferrin receptor, potentiates TNF-induced apoptosis through modulation of the nuclear factor-kappaB signaling pathway. Blood 2007;110:3517-25.
- Nie F, Zhang X, Qi Q, et al. Reactive oxygen species accumulation contributes to gambogic acid-induced apoptosis in human hepatoma SMMC-7721 cells. Toxicology 2009;260:60-7.
- Scholzen T, Gerdes J. The Ki-67 protein: from the known and the unknown. J Cell Physiol 2000;182:311-22.
- Nicholson DW, Thornberry NA. Caspases: killer proteases. Trends Biochem Sci 1997;22:299-306.
- Susin SA, Lorenzo HK, Zamzami N, et al. Molecular characterization of mitochondrial apoptosis-inducing factor. Nature 1999;397:441-6.
- Susnow N, Zeng L, Margineantu D, et al. Bcl-2 family proteins as regulators of oxidative stress. Semin Cancer Biol 2009;19:42-9.
- Yang Y, Yang L, You QD, et al. Differential apoptotic induction of gambogic acid, a novel anticancer natural product, on hepatoma cells and normal hepatocytes. Cancer Lett 2007;256:259-66.
- Gu HY, Guo QL, You QD, et al. Gambogic acid inducing apoptosis in human hepatoma SMMC-7721 cells with p53 and Bax up-regulated. Chin J Nat Med 2005;3:169-171.
- Liu W, Guo QL, You QD, et al. Anticancer effect and apoptosis induction of gambogic acid in human gastric cancer line BGC-823. World J Gastroenterol 2005;11:3655-9.
- Zhao L, Guo QL, You QD, et al. Gambogic acid induces apoptosis and regulates expressions of Bax and Bcl-2 protein in human gastric carcinoma MGC-803 cells. Biol Pharm Bull 2004;27:998-1003.
- Zhao W, Zhou SF, Zhang ZP, et al. Gambogic acid inhibits the growth of osteosarcoma cells in vitro by inducing apoptosis and cell cycle arrest. Oncol Rep 2011;25:1289-95.
- Liu Y, Li W, Ye C, et al. Gambogic acid induces G0/G1 cell cycle arrest and cell migration inhibition via suppressing PDGF receptor β tyrosine phosphorylation and Rac1 activity in rat aortic smooth muscle cells. J Atheroscler Thromb 2010;17:901-13.
- Elmore S. Apoptosis: a review of programmed cell death. Toxicol Pathol 2007;35:495-516.
- Zhang QG, Li CP, Chen JH, et al. Apoptosis-inducing effect of gambogic acid on K562 cells and its mechanism. Zhongguo Shi Yan Xue Ye Xue Za Zhi 2009;17:1443-7.
- Costantini P, Jacotot E, Decaudin D, et al. Mitochondrion as a novel target of anticancer chemotherapy. J Natl Cancer Inst 2000;92:1042-53.
- Zamzami N, Marchetti P, Castedo M, et al. Reduction in mitochondrial potential constitutes an early irreversible step of programmed lymphocyte death in vivo. J Exp Med 1995;181:1661-72.
- Zamzami N, Marchetti P, Castedo M, et al. Sequential reduction of mitochondrial transmembrane potential and generation of reactive oxygen species in early programmed cell death. J Exp Med 1995;182:367-77.
- Ola MS, Nawaz M, Ahsan H. Role of Bcl-2 family proteins and caspases in the regulation of apoptosis. Mol Cell Biochem 2011;351:41-58.
- Adrain C, Creagh EM, Martin SJ. Defying death: showing Bcl-2 the way home. Nat Cell Biol 2003;5:9-11.