Hedgehog signaling pathway and ovarian cancer
Introduction
Epithelial ovarian carcinoma (EOC) is the most common form of ovarian malignancies and the most lethal gynecologic malignancy in the United States, accounting for almost 90% of all ovarian malignant tumors, with approximately 22,000 new cases and 16,000 deaths occurring annually (1,2). It is diagnosed often in advanced stage, owing to the absence of specific signs and clinically significant symptoms and the lack of effective screening programs including appropriate tumor markers, which contributes to the low 5-year relative overall survival of ovarian cancer patients at 46%. As we know, if diagnosed at the localized stage, the 5-year survival rate would be up to 93% (2). To date, the major clinical problems associated with ovarian cancers remain unresolved including malignant progression and rapid emergence of drug resistance. Despite intense scientific research and improved clinical technology, once EOC enters into late stage, even with the extensive surgical debulking and chemotherapy, its prognosis remains dismal (3).
As an important regulatory module during development, the Hedgehog (Hh) signaling pathway can control a variety of developmental processes such as proliferation, differentiation and organogenesis (4). Recently, it has become increasingly clear that in many instances the signaling and molecular players that control development are the same, and when inappropriately regulated, drive tumorigenesis and cancer development (5-13). Here, we discuss the possible involvement of this pathway in the physiologic process and development of cancer in the ovaries. The components of the Hh signaling may provide novel drug targets, which could be explored as crucial combinatorial strategies for the treatment of ovarian cancers.
Constitutive components and transduced mechanisms of Hh signaling
In comparison with Drosophila, in humans, Hh/Gli signaling pathway consists of three ligands, Sonic hedgehog (Shh), Indian hedgehog (Ihh) and Desert hedgehog (Dhh), which can all bind to the 12-pass transmembrane receptor Patch (Ptch) and are expressed at different stages of tumorigenesis in different tissues and may have distinct biological functions (4). When the processed and lipid modified Hh ligand binds to its receptor Ptch, the canonical Hh signal transduction is initiated, resulting in that the inhibitory function of Ptch on the 7-pass transmembrane protein Smoothened (Smo) is abolished and then Smo activates the final arbiter of Hh signaling, the Gli family of transcription factors. Ultimately, the Gli family of Gli1, Gli2 and Gli3, transcriptionally regulates Hh target genes by binding to the specific elements in promoter sequences (14-16). The exact mechanism of signal transduction within the cascade from Smo to Gli proteins is unclear, and the emerging data suggest that primary cilium serves as the processing sites for Gli transcription factors (17), involving a multi-protein complex comprising of a subset of intraflagellar transport proteins, protein kinase A (PKA), glycogen synthase kinase 3β (GSK 3β), casein kinase 1 (CK1), the suppressor of fused (Sufu) and so on (18-21) (Figure 1). Of these, Sufu is thought to play a key role in negatively regulating Hh/Gli signaling, since targeted disruption of the murine Sufu gene leads to the similar phenotype caused by an excess of Hh signaling (22,23).
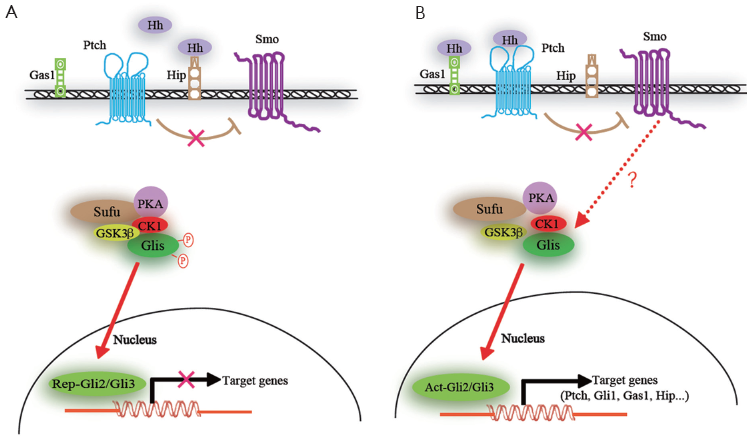
Gli proteins seem to have context-dependent repressor and activator functions. Gli1 functions as the terminal and thus a critical transcriptional activator of the Hh pathway and its function is reinforced by a positive feedback loop as its transcription is induced by Hh signaling (24,25). Gli2 can act as an activator or repressor, whereas Gli3 can serve as a weak activator, mainly functions as a repressor of transcription (26). Gli1 and Gli2 have been shown to have distinct as well as overlapping functions. Three Gli proteins operate together to integrate intercellular Hh signaling and other input, and each can have positive or negative effects (27,28).
Different states of the Gli code can give rise to different or partially overlapping sets of target gene expression profiles, then further facilitate distinct cellular responses (29,30). It is not surprising that deregulation of Hh signaling can cause various diseases including malformations and tumors because of its broad range of direct or indirect targets in different cellular contexts.
Many feedback regulatory mechanisms are employed in Hh signaling pathway. Ptch, Hedgehog interacting protein (Hip), growth arrest specific protein1 (Gas1) and Gli1 are not only the components of the Hh signaling pathway, but also its target genes. Ptch and Hip can negatively regulate the Hh signal, while Gli1 can positively regulate it. Hh signal can downregulate the expression of Gas1, simultaneously, Gas1 functions as the positive regulator of the Hh signal. Aberrant regulations can result in abnormal Hh signal and the expression of specific genes that promote cell proliferation or differentiation.
Hh signaling pathway and carcinogenesis
The Hh signaling pathway is a morphogenesis signaling pathway, which is crucial for the growth and patterning of various tissues during embryonic development (31-36). Cancers occur in various organs after adolescence, suggesting that they are derived from cells harboring mutations that generally occur in genes that control embryonic morphogenesis after the embryonic stage. In the adult, the Hh signaling is significantly reduced, with the remaining activity mostly involved in tissue maintenance and repair, and regulating stem cell behavior in several instances (37,38). Hh signaling has been reported to be reactivated in many types of cancers in ligand-dependent manner (39-42) or ligand-independent manner (5-7,43-45), contributing to carcinogenesis and cancer progression. Whereas the former activation is caused by the overexpressed Hh ligand derived from tumor cells or stroma cells, the later activation is due to mutations of the components within the Hh signaling pathway such as Ptch, Smo and Sufu. Non-canonical Gli activation mediated by epithelial epidermal growth factor receptor (EGFR) or transforming growth factor-β (TGF-β) signaling further activate their downstream targets (46,47) (Figure 2). It therefore renders cancer cells resistant to Smo antagonists.
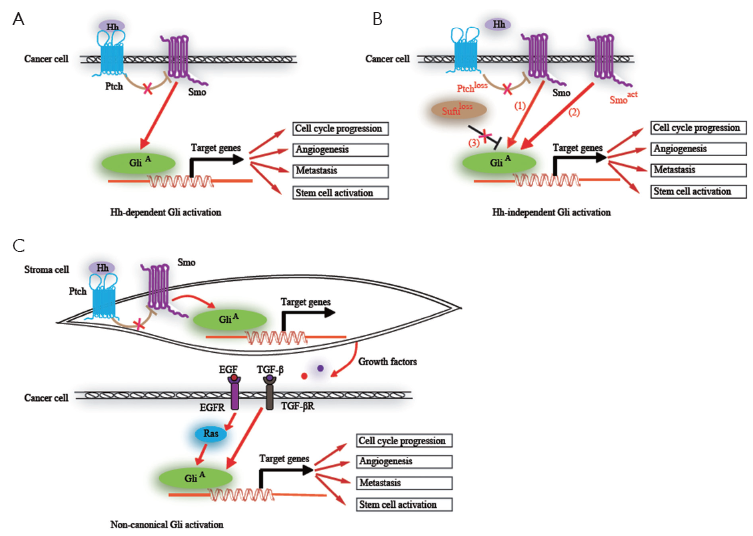
Recent studies of human tumors, such as glioblastoma, pancreatic adenocarcinoma, chronic myeloid leukemia and colon cancer, suggest that Hh signaling regulates cancer stem cell (38,48-52). Both the clonogenicity and tumorigenesis of human cancer stem cells depend on sustained Hh-Gli signaling. It was demonstrated that the Hh signaling components Ptch1, Gli1 and Gli2 are highly expressed in normal human mammary stem/progenitor cells and that these genes are downregulated when differentiation is induced in these cells (53). Thus, the Hh signaling pathway might play a critical role in the continuous self-renewal of tissues from stem cells.
The Hh signaling pathway is known to contribute to cancer invasiveness and metastasis. A report showed that Notch and Shh facilitate neovascularization, angiogenesis, and epithelial-mesenchymal transition (EMT), and contribute to the maintenance of highly-metastatic tumor stem cells (54). Recently, Souzaki M et al. found that the Hh signaling pathway mediates the progression of non-invasive breast cancer to invasive breast cancer (55). It is known that the activation of Hh signaling is involved in the cell cycle progression and invasion of various tumors, including prostate cancer, pancreatic cancer and gastric carcinoma and so on (56-58). Among these, the contribution of Hh pathway to invasiveness was first documented in prostate cancer. As to gastric cancer, the report suggested that Shh signaling promotes motility and invasiveness of it through TGF-β-mediated activation of the Alk5-Smad3 pathway. Combined, these studies suggest that Hh signaling affirmatively contributes to cancer invasion and metastasis.
Cancer therapy targeting hedgehog signaling
Because of the widespread involvement of the Hh pathway in cancers that controls fundamental cellular processes such as cell growth and death, the promising therapeutic strategies against cancers may be developed through the modulation of the Hh pathway in the near future. Several approaches to block this pathway are under development. Sustained application of specific Hh pathway inhibitors has been proven effective in preventing growth of many tumors in vitro (49,59) and in xenografts (60,61).
Small molecular modulators of Hh signaling have been an intense interest in recent years, and the last few years have brought a significant increase in the identification of related inhibitors (62-66). The natural product alkaloid cyclopamine was one of the first small-molecule inhibitors of the Hh pathway to be reported (67). To date, the majority of reported Hh pathway inhibitors target Smo including cyclopamine, IPI-926, GDC-0449, BMS-833923 and so on, and several have advanced to human clinical trials, which have different molecular mechanisms respectively. The impact of terminating Hh signaling at the level of Gli genes on the tumorigenesis is little known. Few agents are available that target Gli genes, which constitute the final step in the Hh pathway, whereas, GANT61 was identified as a more effective small molecular inhibitor of Gli in comparison with Smo, which induced extensive cell death or apoptosis in cancer cell lines in vitro and prohibited additional tumor growth in the xenograft assays through inhibiting the expression of the target genes downstream of Gli (10,60). More recently, as the first reported inhibitor of Shh, robotnikinin was identified to bind to the transmembrane receptor Ptch, reversing its inhibitory effect on Smo (68,69). Collectively, these Hh signaling inhibitors have brought about the expectation that the Hh signaling pathway could provide effective approaches for cancer therapy. In order to implement the individualized therapy with all Hh pathway-dependent cancers, we must select the appropriate inhibitors corresponding to the molecular mechanism of altered Hh pathway.
Hh signaling pathway and ovarian cancer
Hh signaling has not been found in the mature vertebrate ovary (70), while it was identified to act specifically on the stem cells in Drosophila ovary (71). These stem cells are responsible for the regulated repair of the surface epithelium after the ovulatory rupture (72). Under abnormal conditions that lead to enhanced Hh signaling, these stem cells might ultimately transform into cancer stem cells and lead to malignant progression (73,74).
It is generally accepted that ovarian epithelial cancer derives from ovarian surface epithelium (OSE) that covers the ovary. As we know, by comparison with mesothelia elsewhere, OSE is a simple, rather primitive mesothelium with both epithelial and mesenchymal characteristics, which retains the properties of relatively uncommitted pluripotent cells and has the ability to differentiate diversely in response to different stimuli (75). Apart from other cancers, OSE becomes more committed to an epithelial phenotype in association with increased E-cadherin expression (75,76) when it progresses to malignancy, through adherens junction mediated by E-cadherin, tumor cells can often aggregate and form spheroid-like structures that followed by implanting and invading into intra-abdominal tissues (76-78). Ray A et al. demonstrated that Hh signaling pathway can regulate the growth of ovarian cancer spheroid forming cells, which have the properties of cancer stem cells of self-renewal, differentiation, and chemoresistance (79).
Recently, aberrant activation of Hh signaling pathway has been reported in ovarian cancers (80). In this report, the authors indicated that Hh signaling pathway is activated in ovarian carcinomas, concerning cell proliferation, while, its inhibition precipitates growth suppression and cancer cells apoptosis. They found that Dhh expression was correlated with poor prognosis of patients with ovarian carcinoma. Bhattacharya R et al. found that the upregulation of the Hh pathway was present in primary ovarian tumors and all of the human ovarian cancer cell lines tested. They proposed that the Hh pathway can help maintain the clonal growth of human ovarian carcinoma-derived cell lines (81). Liao X et al. showed for the first time that overexpression of Gli1 and Patched was correlated with poor clinical outcome in ovarian cancers, which provided a molecular basis for the role of Hh pathway in ovarian cancers (82). In contrast to previous reports, Yang L et al. documented that they only detected a small proportion of ovarian cancers with Hh signaling target gene expression. They suggested that activation of the Hh pathway would not be frequent in ovarian cancers (83). Schmid S et al. found that a subset of patients with ovarian cancer (25%) in their study had increased expression of the Hh signaling and transcription factors Gli2 (84). The published data concerning the Hh signaling pathway in ovarian cancer are contradictory, which is probably resulted from the cross talk between Hh and other signaling pathways.
As mentioned above, abnormal Hh signaling plays important roles in the development and progression of ovarian cancers, which has been further verified through some in vitro and in vivo studies. Accordingly, the inhibition of Hh pathway might be a valid therapeutic strategy for ovarian cancers. Treatment with a monoclonal antibody against Shh resulted in a dose-dependent decrease in cell proliferation (81). Similarly, treatment of cultured ovarian cancer cells with the Smo inhibitor cyclopamine has been found to induce cell cycle arrest in G1 and promote apoptosis (80). McCann CK et al. showed that inhibition of Hh signaling by the inhibitor IPI-926, a derivative of cyclopamine with increased oral bioavailability and a longer half-life, antagonized serous ovarian cancer growth in a primary xenograft model (85). In addition, ovarian carcinoma cell lines-derived xenografts are also susceptible to Hh pathway inhibition as their growth is markedly impaired following treatment with cyclopamine (81). To date, all studies that determine the effect of the Hh inhibitors on growth and progression of ovarian cancer were targeted to Smo. Less is known about whether the therapeutic approaches targeted to downstream genes of Smo might be more promising.
Prospect
Significant progress has been made in understanding the pathogenesis and targeted therapy of ovarian cancer, especially in last 5 years. Cognition on the genetics and Hh pathways in both epithelial cells and stromal cells at the molecular levels allows us to interfere with ovarian cancers using defined pathway inhibitor through the in vitro and in vivo assays. Further dissection of the role of Hh pathway in the initiation and progression of ovarian carcinoma will create new drug targets for its therapeutic intervention.
Acknowledgements
This work was supported by Grants from the National Natural Science Foundation of China (No. 31171359) and the Ministry of Science and Technology of China (No. 2010CB535001).
Disclosure: The authors declare no conflict interest.
References
- Dinulescu DM, Ince TA, Quade BJ, et al. Role of K-ras and Pten in the development of mouse models of endometriosis and endometrioid ovarian cancer. Nat Med 2005;11:63-70. [PubMed]
- Jemal A, Siegel R, Ward E, et al. Cancer statistics, 2009. CA Cancer J Clin 2009;59:225-49. [PubMed]
- Rosen DG, Yang G, Liu G, et al. Ovarian cancer: pathology, biology, and disease models. Front Biosci 2009;14:2089-102. [PubMed]
- Ingham PW, McMahon AP. Hedgehog signaling in animal development: paradigms and principles. Genes Dev 2001;15:3059-87. [PubMed]
- Kawabata N, Ijiri K, Ishidou Y, et al. Pharmacological inhibition of the Hedgehog pathway prevents human rhabdomyosarcoma cell growth. Int J Oncol 2011;39:899-906. [PubMed]
- Grammel D, Warmuth-Metz M, von Bueren AO, et al. Sonic hedgehog-associated medulloblastoma arising from the cochlear nuclei of the brainstem. Acta Neuropathol 2012;123:601-14. [PubMed]
- Wang X, Venugopal C, Manoranjan B, et al. Sonic hedgehog regulates Bmi1 in human medulloblastoma brain tumor-initiating cells. Oncogene 2012;31:187-99. [PubMed]
- Nagao H, Ijiri K, Hirotsu M, et al. Role of GLI2 in the growth of human osteosarcoma. J Pathol 2011;224:169-79. [PubMed]
- Das S, Samant RS, Shevde LA. The hedgehog pathway conditions the bone microenvironment for osteolytic metastasis of breast cancer. Int J Breast Cancer 2012;2012:298623.
- Mazumdar T, DeVecchio J, Agyeman A, et al. The GLI genes as the molecular switch in disrupting Hedgehog signaling in colon cancer. Oncotarget 2011;2:638-45. [PubMed]
- Chang HH, Chen BY, Wu CY, et al. Hedgehog overexpression leads to the formation of prostate cancer stem cells with metastatic property irrespective of androgen receptor expression in the mouse model. J Biomed Sci 2011;18:6. [PubMed]
- Eberl M, Klingler S, Mangelberger D, et al. Hedgehog-EGFR cooperation response genes determine the oncogenic phenotype of basal cell carcinoma and tumour-initiating pancreatic cancer cells. EMBO Mol Med 2012;4:218-33. [PubMed]
- Caro I, Low JA. The role of the hedgehog signaling pathway in the development of basal cell carcinoma and opportunities for treatment. Clin Cancer Res 2010;16:3335-9. [PubMed]
- Katoh Y, Katoh M. Hedgehog target genes: mechanisms of carcinogenesis induced by aberrant hedgehog signaling activation. Curr Mol Med 2009;9:873-86. [PubMed]
- Kasper M, Regl G, Frischauf AM, et al. GLI transcription factors: mediators of oncogenic Hedgehog signalling. Eur J Cancer 2006;42:437-45. [PubMed]
- Thiyagarajan S, Bhatia N, Reagan-Shaw S, et al. Role of GLI2 transcription factor in growth and tumorigenicity of prostate cells. Cancer Res 2007;67:10642-6. [PubMed]
- Rohatgi R, Milenkovic L, Scott MP. Patched1 regulates hedgehog signaling at the primary cilium. Science 2007;317:372-6. [PubMed]
- Kasper M, Jaks V, Fiaschi M, et al. Hedgehog signalling in breast cancer. Carcinogenesis 2009;30:903-11. [PubMed]
- Huangfu D, Liu A, Rakeman AS, et al. Hedgehog signalling in the mouse requires intraflagellar transport proteins. Nature 2003;426:83-7. [PubMed]
- Huangfu D, Anderson KV. Cilia and Hedgehog responsiveness in the mouse. Proc Natl Acad Sci U S A 2005;102:11325-30. [PubMed]
- Varjosalo M, Taipale J. Hedgehog signaling. J Cell Sci 2007;120:3-6. [PubMed]
- Kogerman P, Grimm T, Kogerman L, et al. Mammalian suppressor-of-fused modulates nuclear-cytoplasmic shuttling of Gli-1. Nat Cell Biol 1999;1:312-9. [PubMed]
- Svärd J, Heby-Henricson K, Persson-Lek M, et al. Genetic elimination of Suppressor of fused reveals an essential repressor function in the mammalian Hedgehog signaling pathway. Dev Cell 2006;10:187-97. [PubMed]
- Bai CB, Stephen D, Joyner AL. All mouse ventral spinal cord patterning by hedgehog is Gli dependent and involves an activator function of Gli3. Dev Cell 2004;6:103-15. [PubMed]
- Stecca B, Ruiz I, Altaba A. Context-dependent regulation of the GLI code in cancer by HEDGEHOG and non-HEDGEHOG signals. J Mol Cell Biol 2010;2:84-95. [PubMed]
- Stamataki D, Ulloa F, Tsoni SV, et al. A gradient of Gli activity mediates graded Sonic Hedgehog signaling in the neural tube. Genes Dev 2005;19:626-41. [PubMed]
- Nguyen V, Chokas AL, Stecca B, et al. Cooperative requirement of the Gli proteins in neurogenesis. Development 2005;132:3267-79. [PubMed]
- Tyurina OV, Guner B, Popova E, et al. Zebrafish Gli3 functions as both an activator and a repressor in Hedgehog signaling. Dev Biol 2005;277:537-56. [PubMed]
- Vokes SA, Ji H, McCuine S, et al. Genomic characterization of Gli-activator targets in sonic hedgehog-mediated neural patterning. Development 2007;134:1977-89. [PubMed]
- Vokes SA, Ji H, Wong WH, et al. A genome-scale analysis of the cis-regulatory circuitry underlying sonic hedgehog-mediated patterning of the mammalian limb. Genes Dev 2008;22:2651-63. [PubMed]
- Duench K, Franz-Odendaal TA. BMP and Hedgehog signaling during the development of scleral ossicles. Dev Biol 2012;365:251-8. [PubMed]
- Laufer E, Kesper D, Vortkamp A, et al. Sonic hedgehog signaling during adrenal development. Mol Cell Endocrinol 2012;351:19-27. [PubMed]
- Merchant JL. Hedgehog signalling in gut development, physiology and cancer. J Physiol 2012;590:421-32. [PubMed]
- Migone FF, Ren Y, Cowan RG, et al. Dominant activation of the hedgehog signaling pathway alters development of the female reproductive tract. Genesis 2012;50:28-40. [PubMed]
- Nat R, Salti A, Suciu L, et al. Pharmacological modulation of the Hedgehog pathway differentially affects dorsal/ventral patterning in mouse and human embryonic stem cell models of telencephalic development. Stem Cells Dev 2012;21:1016-46. [PubMed]
- Ren Y, Cowan RG, Migone FF, et al. Overactivation of hedgehog signaling alters development of the ovarian vasculature in mice. Biol Reprod 2012;86:174. [PubMed]
- Ihrie RA, Shah JK, Harwell CC, et al. Persistent sonic hedgehog signaling in adult brain determines neural stem cell positional identity. Neuron 2011;71:250-62. [PubMed]
- Tang SN, Fu J, Nall D, et al. Inhibition of sonic hedgehog pathway and pluripotency maintaining factors regulate human pancreatic cancer stem cell characteristics. Int J Cancer 2012;131:30-40. [PubMed]
- Yauch RL, Dijkgraaf GJ, Alicke B, et al. Smoothened mutation confers resistance to a Hedgehog pathway inhibitor in medulloblastoma. Science 2009;326:572-4. [PubMed]
- Reifenberger J, Wolter M, Knobbe CB, et al. Somatic mutations in the PTCH, SMOH, SUFUH and TP53 genes in sporadic basal cell carcinomas. Br J Dermatol 2005;152:43-51. [PubMed]
- Taylor MD, Liu L, Raffel C, et al. Mutations in SUFU predispose to medulloblastoma. Nat Genet 2002;31:306-10. [PubMed]
- Byrom J, Mudaliar V, Redman CW, et al. Loss of heterozygosity at chromosome 9q22-31 is a frequent and early event in ovarian tumors. Int J Oncol 2004;24:1271-7. [PubMed]
- Yamasaki A, Kameda C, Xu R, et al. Nuclear factor kappaB-activated monocytes contribute to pancreatic cancer progression through the production of Shh. Cancer Immunol Immunother 2010;59:675-86. [PubMed]
- Yauch RL, Gould SE, Scales SJ, et al. A paracrine requirement for hedgehog signalling in cancer. Nature 2008;455:406-10. [PubMed]
- Tian H, Callahan CA, DuPree KJ, et al. Hedgehog signaling is restricted to the stromal compartment during pancreatic carcinogenesis. Proc Natl Acad Sci U S A 2009;106:4254-9. [PubMed]
- Allegra CJ, Aberle DR, Ganschow P, et al. National Institutes of Health State-of-the-Science Conference statement: Diagnosis and Management of Ductal Carcinoma In Situ September 22-24, 2009. J Natl Cancer Inst 2010;102:161-9. [PubMed]
- Ruiz i Altaba A, Mas C, Stecca B. The Gli code: an information nexus regulating cell fate, stemness and cancer. Trends Cell Biol 2007;17:438-47. [PubMed]
- Merchant AA, Matsui W. Targeting Hedgehog--a cancer stem cell pathway. Clin Cancer Res 2010;16:3130-40. [PubMed]
- Singh BN, Fu J, Srivastava RK, et al. Hedgehog signaling antagonist GDC-0449 (Vismodegib) inhibits pancreatic cancer stem cell characteristics: molecular mechanisms. PLoS One 2011;6:e27306. [PubMed]
- Varnat F, Duquet A, Malerba M, et al. Human colon cancer epithelial cells harbour active HEDGEHOG-GLI signalling that is essential for tumour growth, recurrence, metastasis and stem cell survival and expansion. EMBO Mol Med 2009;1:338-51. [PubMed]
- Bar EE, Chaudhry A, Lin A, et al. Cyclopamine-mediated hedgehog pathway inhibition depletes stem-like cancer cells in glioblastoma. Stem Cells 2007;25:2524-33. [PubMed]
- Zhao C, Chen A, Jamieson CH, et al. Hedgehog signalling is essential for maintenance of cancer stem cells in myeloid leukaemia. Nature 2009;458:776-9. [PubMed]
- Liu S, Dontu G, Mantle ID, et al. Hedgehog signaling and Bmi-1 regulate self-renewal of normal and malignant human mammary stem cells. Cancer Res 2006;66:6063-71. [PubMed]
- Bailey JM, Singh PK, Hollingsworth MA. Cancer metastasis facilitated by developmental pathways: Sonic hedgehog, Notch, and bone morphogenic proteins. J Cell Biochem 2007;102:829-39. [PubMed]
- Souzaki M, Kubo M, Kai M, et al. Hedgehog signaling pathway mediates the progression of non-invasive breast cancer to invasive breast cancer. Cancer Sci 2011;102:373-81. [PubMed]
- Yoo YA, Kang MH, Kim JS, et al. Sonic hedgehog signaling promotes motility and invasiveness of gastric cancer cells through TGF-beta-mediated activation of the ALK5-Smad 3 pathway. Carcinogenesis 2008;29:480-90. [PubMed]
- Karhadkar SS, Bova GS, Abdallah N, et al. Hedgehog signalling in prostate regeneration, neoplasia and metastasis. Nature 2004;431:707-12. [PubMed]
- Onishi H, Kai M, Odate S, et al. Hypoxia activates the hedgehog signaling pathway in a ligand-independent manner by upregulation of Smo transcription in pancreatic cancer. Cancer Sci 2011;102:1144-50. [PubMed]
- Gould A, Missailidis S. Targeting the hedgehog pathway: the development of cyclopamine and the development of anti-cancer drugs targeting the hedgehog pathway. Mini Rev Med Chem 2011;11:200-13. [PubMed]
- Lauth M, Bergström A, Shimokawa T, et al. Inhibition of GLI-mediated transcription and tumor cell growth by small-molecule antagonists. Proc Natl Acad Sci U S A 2007;104:8455-60. [PubMed]
- Dierks C, Beigi R, Guo GR, et al. Expansion of Bcr-Abl-positive leukemic stem cells is dependent on Hedgehog pathway activation. Cancer Cell 2008;14:238-49. [PubMed]
- Chenna V, Hu C, Pramanik D, et al. A polymeric nanoparticle encapsulated small-molecule inhibitor of Hedgehog signaling (NanoHHI) bypasses secondary mutational resistance to Smoothened antagonists. Mol Cancer Ther 2012;11:165-73. [PubMed]
- LoRusso PM, Rudin CM, Reddy JC, et al. Phase I trial of hedgehog pathway inhibitor vismodegib (GDC-0449) in patients with refractory, locally advanced or metastatic solid tumors. Clin Cancer Res 2011;17:2502-11. [PubMed]
- Tian F, Schrödl K, Kiefl R, et al. The hedgehog pathway inhibitor GDC-0449 alters intracellular Ca2+ homeostasis and inhibits cell growth in cisplatin-resistant lung cancer cells. Anticancer Res 2012;32:89-94. [PubMed]
- Warzecha J, Dinges D, Kaszap B, et al. Effect of the Hedgehog-inhibitor cyclopamine on mice with osteosarcoma pulmonary metastases. Int J Mol Med 2012;29:423-7. [PubMed]
- Peukert S, Miller-Moslin K. Small-molecule inhibitors of the hedgehog signaling pathway as cancer therapeutics. ChemMedChem 2010;5:500-12. [PubMed]
- Cooper MK, Porter JA, Young KE, et al. Teratogen-mediated inhibition of target tissue response to Shh signaling. Science 1998;280:1603-7. [PubMed]
- Kiselyov AS. Targeting the hedgehog signaling pathway with small molecules. Anticancer Agents Med Chem 2006;6:445-9. [PubMed]
- Stanton BZ, Peng LF, Maloof N, et al. A small molecule that binds Hedgehog and blocks its signaling in human cells. Nat Chem Biol 2009;5:154-6. [PubMed]
- Bitgood MJ, Shen L, McMahon AP. Sertoli cell signaling by Desert hedgehog regulates the male germline. Curr Biol 1996;6:298-304. [PubMed]
- Zhang Y, Kalderon D. Hedgehog acts as a somatic stem cell factor in the Drosophila ovary. Nature 2001;410:599-604. [PubMed]
- Bukovsky A, Gupta SK, Virant-Klun I, et al. Study origin of germ cells and formation of new primary follicles in adult human and rat ovaries. Methods Mol Biol 2008;450:233-65. [PubMed]
- Burgos-Ojeda D, Rueda BR, Buckanovich RJ. Ovarian cancer stem cell markers: prognostic and therapeutic implications. Cancer Lett 2012;322:1-7. [PubMed]
- Cao L, Shao M, Schilder J, et al. Tissue transglutaminase links TGF-β, epithelial to mesenchymal transition and a stem cell phenotype in ovarian cancer. Oncogene 2012;31:2521-34. [PubMed]
- Auersperg N, Wong AS, Choi KC, et al. Ovarian surface epithelium: biology, endocrinology, and pathology. Endocr Rev 2001;22:255-88. [PubMed]
- Ahmed N, Thompson EW, Quinn MA. Epithelial-mesenchymal interconversions in normal ovarian surface epithelium and ovarian carcinomas: an exception to the norm. J Cell Physiol 2007;213:581-8. [PubMed]
- Wu C, Cipollone J, Maines-Bandiera S, et al. The morphogenic function of E-cadherin-mediated adherens junctions in epithelial ovarian carcinoma formation and progression. Differentiation 2008;76:193-205. [PubMed]
- Symowicz J, Adley BP, Gleason KJ, et al. Engagement of collagen-binding integrins promotes matrix metalloproteinase-9-dependent E-cadherin ectodomain shedding in ovarian carcinoma cells. Cancer Res 2007;67:2030-9. [PubMed]
- Ray A, Meng E, Reed E, et al. Hedgehog signaling pathway regulates the growth of ovarian cancer spheroid forming cells. Int J Oncol 2011;39:797-804. [PubMed]
- Chen X, Horiuchi A, Kikuchi N, et al. Hedgehog signal pathway is activated in ovarian carcinomas, correlating with cell proliferation: it's inhibition leads to growth suppression and apoptosis. Cancer Sci 2007;98:68-76. [PubMed]
- Bhattacharya R, Kwon J, Ali B, et al. Role of hedgehog signaling in ovarian cancer. Clin Cancer Res 2008;14:7659-66. [PubMed]
- Liao X, Siu MK, Au CW, et al. Aberrant activation of hedgehog signaling pathway in ovarian cancers: effect on prognosis, cell invasion and differentiation. Carcinogenesis 2009;30:131-40. [PubMed]
- Yang L, He J, Huang S, et al. Activation of hedgehog signaling is not a frequent event in ovarian cancers. Mol Cancer 2009;8:112. [PubMed]
- Schmid S, Bieber M, Zhang F, et al. Wnt and hedgehog gene pathway expression in serous ovarian cancer. Int J Gynecol Cancer 2011;21:975-80. [PubMed]
- McCann CK, Growdon WB, Kulkarni-Datar K, et al. Inhibition of Hedgehog signaling antagonizes serous ovarian cancer growth in a primary xenograft model. PLoS One 2011;6:e28077. [PubMed]