Human endogenous retroviral syncytin exerts inhibitory effect on invasive phenotype of B16F10 melanoma cells
Introduction
Syncytin is a human endogenous retroviral (HERV) envelope protein with fusogenic properties and plays an important role in the formation of syncytiotrophoblasts in human placenta (1-4). Probably due to its critical role in the genesis of placenta (5,6), the syncytin encoding gene is highly conserved, unlike most of the other HERV sequences (7). Apart from its expression in placenta, syncytin has also been found to be expressed in testis, brain and osteoclasts (8-10). Shortly after the discovery of the syncytin gene, a closely related HERV gene, which is later named as syncytin-2 (thus the original one is also named as syncytin-1), is identified (11,12). Both of the genes are found to be expressed in human placenta and their products on cell surfaces have the same fusogenic capability (11-13). In mediating cell-cell fusion, syncytin uses neutral amino acid transporter 2 (ASCT-2, also a retroviral receptor) as the main receptor and ASCT-1 as an auxiliary receptor (14), while syncytin-2 uses an assumed carbohydrate transporter, namely major facilitator superfamily domain-containing protein 2 (MFSD-2), as its receptor (11).
Apart from its physiological function in placenta, the role of syncytin in cancers has been investigated recently (15-18). Syncytin is expressed in human breast cancer (15), endothelial carcinoma (17), as well as leukemia cells (19). Animal models have shown that syncytin is involved in both cancer to cancer and cancer to endothelial cell fusions (15). Yet, the role and prognostic impact of syncytin expressed in tumor cells are still controversial. Some studies have indicated that syncytin expression in human endometrial tumors implies that the tumor cells are more malignant (17), whereas other studies have shown that syncytin may also serve as a positive prognostic factor in human breast cancers (20). Therefore, further research is needed to clarify the roles of syncytin in cancer cells.
In this study, we investigated whether syncytin expression could alter the phenotype of tumor cells using syncytin-expressing B16F10 subline cells as a model. Our results indicated that the invasion potential of the tumor cells was significantly suppressed by syncytin expression. These results suggest that syncytin expression in some cancers may confine metastasis and thus serve as a positive prognostic factor.
Materials and methods
Reagents
Eukaryotic expression vector pEGFP-N1 was obtained from ClonTech (Palo Alto, CA, USA). The transfection reagent Trans-EZ was a product of Shanghai Sunbio Medical Biotechnology Co. (Shanghai, China). DNA marker DL5000, Xho I, EcoR I, T4 DNA ligase, and high fidelity PrimeSTAR HS DNA polymerase were purchased from TaKaRa (Dalian, China). Propidium iodide (PI) was from Sigma-Aldrich (St. Louis, MO, USA). RNase A was purchased from Invitrogen (Carlsbad, CA, USA).
Cell culture
Mouse B16F10 cell line was obtained from the Cell Bank of the Chinese Academy of Sciences (Shanghai, China), cultured in DMEM (Invitrogen) supplemented with 10% fetal bovine serum (Invitrogen), 100 U/mL penicillin and 100 µg/mL streptomycin (Invitrogen) and maintained at 37 °C in a humidified incubator of 5% CO2.
Extraction of total RNA
Total RNA was extracted by TRIzol reagent (Invitrogen) from each sample according to the manufacturer’s instruction and RNA quality was judged by agarose gel electrophoresis. After concentration calculation by ultraviolet (UV) photometry, the total RNA products were immediately transcribed into cDNAs using a PrimeScriptTM 1st Strand cDNA Synthesis Kit (Takara Biotechnology, China).
Cloning and sequencing of syncytin
Polymerase chain reaction (PCR) was performed to amplify the human syncytin cDNA using high-fidelity DNA polymerase according the provided protocol. The forward primer 5'-CGCGCTCGAGGCCAccATGGCCCTCCCTTATCATATTTTTCTCTTTA CTG-3' (containing an Xho I site) and reverse primer 5'-AATCGAATTCGACTGCTTCCTGCTGAATTGG-3' (containing an EcoR I site) were used to amplify human syncytin cDNA. The following PCR program was used for cDNA amplification: initial denaturation at 94 °C for 2 min, 35 cycles of 94 °C for 30 s, 55 °C for 30 s and 72 °C for 2 min, and a final extension at 72 °C for 10 min. The PCR products of 1.65 kb were excised after agarose gel electrophoresis and purified by QIAquick Gel Extraction Kit (QIAGEN, Germany). The purified PCR products were ligated into pEGFP-N1 vector after being digested with Xho I and EcoR I. Ligations were transformed into Escherichia coli DH5α and 10 bacterial colonies were picked from per primer pair and incubated with shaking overnight in 5 mL LB medium containing 34 µg/mL kanamycin. The plasmids that contained the desired inserts were identified by double restriction enzyme digestion with Xho I and EcoR I, and DNA sequencing performed by Invitrogen (Guangzhou, China).
Stable transfection of pEGFP-N1 and pEGFP/syncytin
The plasmid encoding the syncytin-EGFP fusion protein and pEGFP-N1 vector were transfected into B16F10 cells by Trans-EZ according to the manufacturer’s protocol in a 24-well plate, respectively. The cells were further cultured for 48 h in a humidified 5% CO2 incubator at 37 °C. The expression of transfected genes was revealed by EGFP fluorescence under an inverted confocal microscope (Nikon, Japan). Transfected B16F10 cells were cultured with selection medium containing 800 µg/mL of G418 for 14 d and stable clones were isolated and maintained under 400 µg/mL of G418 selection pressure. Before each experiment, the cells were incubated under normal medium without G418 for 24 h.
Measurement of cell proliferation and viability
The proliferation of B16F10 cells was measured using 3-(4,5-dimethylthiazol-2-yl)-5-(3-carboxymethoxyphenyl)-2-(4-sulfophenyl)-2H-tetrazolium (MTS) assay (CellTiter 96 Aqueous One Solution kit; Promega, Madison, WI). The cells were seeded into 96-well plates at 500, 1,000, 2,000, and 4,000 cells per well (100 µL). After incubation further for 48 h, MTS (20 µL) was added to each well and incubated at 37 °C for 1 to 4 h. The absorbance at 490 nm was measured using a microplate reader (Model 680; Bio-Rad, Richmond, CA, USA). Three independent experiments were performed, each in triplicates.
Cell cycle analysis
Analysis of cell cycle was performed as described previously (21). In brief, B16F10 cells were fixed and stained with PBS containing 50 µg/mL PI and 30 µg/mL of RNase A. DNA content data were acquired using a flow cytometer (FACSCalibur; Becton Dickinson, Mountain View, CA, USA) and analyzed with CELLQuest software (Becton Dickinson).
Cell migration assay
The scratch wound-healing assay was performed to determine cell migration ability as described previously (22). Cells were photographed under microscope at 0 and 24 h.
Matrigel invasion assay
The in vitro invasion assay was performed as described previously (22). In brief, cells were added to the Matrigel-coated upper chambers of Transwell (Corning, Cambridge, MA) at 1×104 in 100 µL and the chambers were placed into a 24-well plate containing complete medium. After incubation for 48 h, the cells on the upper membrane surface were gently removed and the lower membrane surfaces were stained with 0.1% crystal violet in 20% methanol. Stained cells were photographed under a Nikon microscope (Nikon, Japan).
Immunofluorescent microscopy
B16F10 cells were seeded on cover slip in 6-well plate and incubated at 37 °C overnight. The cells were washed with PBS containing 3% fetal calf serum (FCS) and fixed with cold acetone. After brief washing, the cells were incubated with rabbit-anti-human syncytin antibody (Santa Cruz Biotechnology Inc., Santa Cruz, CA) at room temperature for 2 h. After washing with PBS-3% FCS, phycoerythrin (PE)-labeled goat-anti-rabbit IgG (Dako, Denmark) was added and incubated for 1 h. The subcellular distribution of the expressed syncytin-EGFP fusion proteins after PE fluorescent staining was revealed by an inverted fluorescent microscope (Nikon).
Western blot analysis
Cell lysates were prepared by lysing the PBS-washed cells with RIPA buffer (Beyotime, Haimen, China). Protein concentration was determined by bicinchoninic acid (BCA) reagents (Pierce, Rockford, IL, USA). Total proteins (40 µg) were separated by sodium dodecyl sulfate-polyacrylamide gel electrophoresis (SDS-PAGE) followed by electro-transfer to polyvinylidene difluoride membrane. The membranes were immunoblotted with rabbit-anti-human syncytin antibody (Santa Cruz) or anti-glyceraldehyde-3-phosphate dehydrogenase (GAPDH) antibody (Cell Signaling Technologies; Danvers, MA, USA) and horseradish peroxidase (HRP)-conjugated second antibodies (Jackson ImmunoResearch; West Grove, PA, USA). Bands were revealed by a BeyoECL Plus kit (Beyotime) and recorded on X-ray films (Kodak, Xiamen, China). Images were acquired by FluorChem 8000 imaging system (AlphaInnotech, San Leandro, CA, USA).
Statistical analysis
Data are expressed as x̄±s (n=3). Statistical analysis was performed using GraphPad Prism 4.0 (GraphPad Software Inc., San Diego, CA). Student’s t-test was used to analyze the statistical significance between two groups. P<0.05 was considered statistically significant.
Results
Construction of syncytin-EGFP eukaryotic expression plasmid
The cDNA encoding human syncytin was amplified by PCR from the total RNA of a human placenta sample. The DNA fragments with the expected length (1,641 bp) were inserted into pEGFP-N1, and three independently transformed DH5α clones were identified to have the correct insert (Figure 1, lanes 3-5). DNA sequencing of these clones confirmed that they all contained the right sequence of human syncytin (designated pEGFP/Syncytin). The cDNA sequence for human syncytin has been submitted to GenBank (accession number: GQ919057).
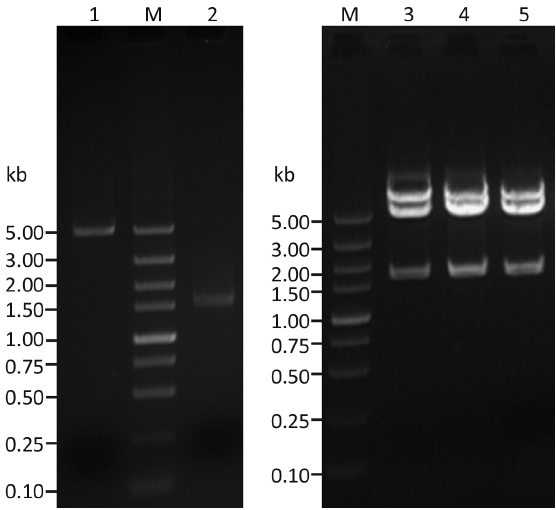
Establishment of B16F10 sublines that stably expressed syncytin-EGFP fusion protein and EGFP
pEGFP/Syncytin and pEGFP-N1 were transfected into B16F10 cells, respectively. Sublines of B16F10 cells that stably expressed EGFP or syncytin-EGFP were isolated by G418 selection and named as B16F10/EGFP and B16F10/Syncytin, respectively (Figure 2A,B). Fluorescent microscopy showed that EGFP was distributed evenly in both the B16F10/EGFP and B16F10/Syncytin cells, and also in their subcultures (Figure 2), indicating that EGFP or syncytin-EGFP fusion proteins were stably expressed in these cells. Expression of syncytin-EGFP fusion protein in B16F10/syncytin cells, instead of in B16F10/EGFP cells, was further confirmed by immunofluorescent staining with an anti-human syncytin antibody and followed by a PE-conjugated second antibody (Figure 3A-F). Western blotting analysis using syncytin-specific antibody also revealed that the syncytin-EGFP fusion protein was specifically expressed in B16F10/Syncytin cells with an apparent molecular weight of 89 kD (Figure 3G). These results indicated that syncytin-EGFP or EGFP proteins were stably expressed in the isolated sublines of B16F10 cells, respectively.
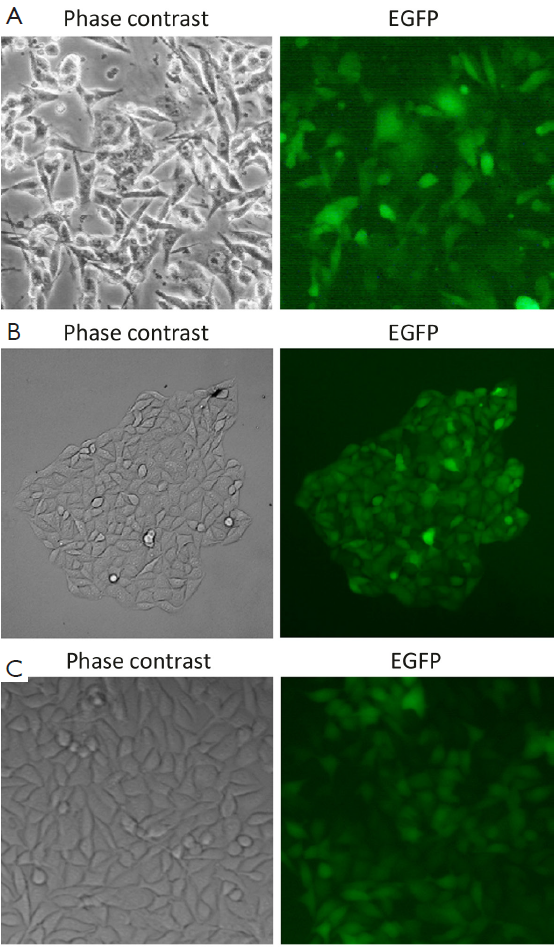
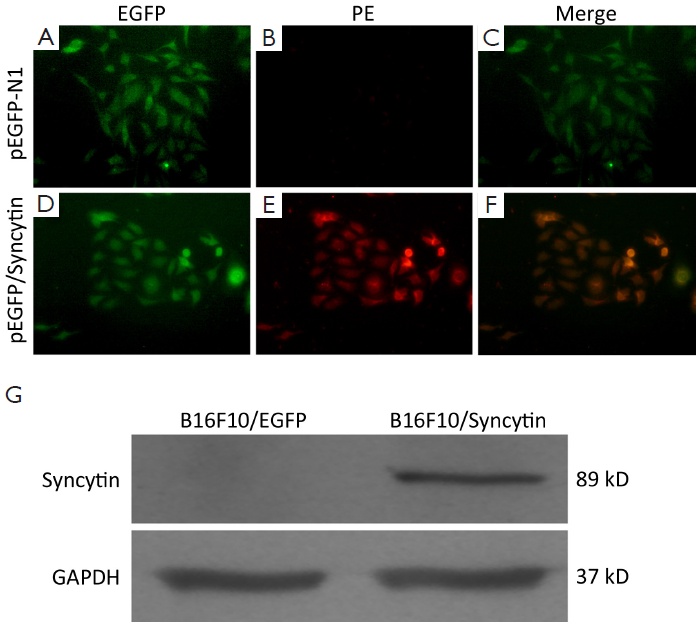
Characterization of B16F10 cells expressing syncytin-EGFP fusion protein
Next, we examined the characteristics of B16F10/EGFP and B16F10/Syncytin cells, including cell proliferation rates, cell cycle distribution and invasive phenotypes. Cell proliferation rates were detected by MTS assay. The results showed that the proliferation rate of B16F10/EGFP cells was nearly doubled as compared with that of B16F10/Syncytin cells (Figure 4). Cell cycle analysis indicated that B16F10/Syncytin cells had a slight decrease of the S and G2/M phase ratios but an increase of G1 phase proportion when compared with B16F10/EGFP cells (Figure 5). The ratio of multinucleated cells (with >4N DNA contents) was also increased in B16F10/Syncytin cells (Figure 5), which was further confirmed by cell morphology analysis (Figure 6). The multinucleated cells had as many as 5 nuclei (Figure 6C). In addition, the cells expressing syncytin-EGFP adhered together more tightly than control cells without syncytin. One additional clone that expressed syncytin-EGFP had similar phenotypes of morphology and multinucleation (data not shown). These results suggest that the syncytin-EGFP fusion protein retains the fusogenic activity of syncytin in B16F10 cells.
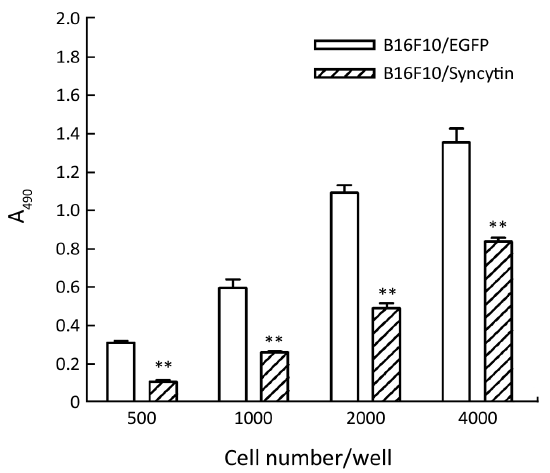
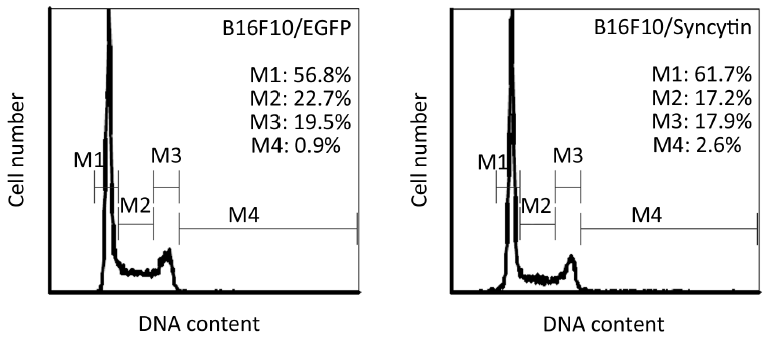
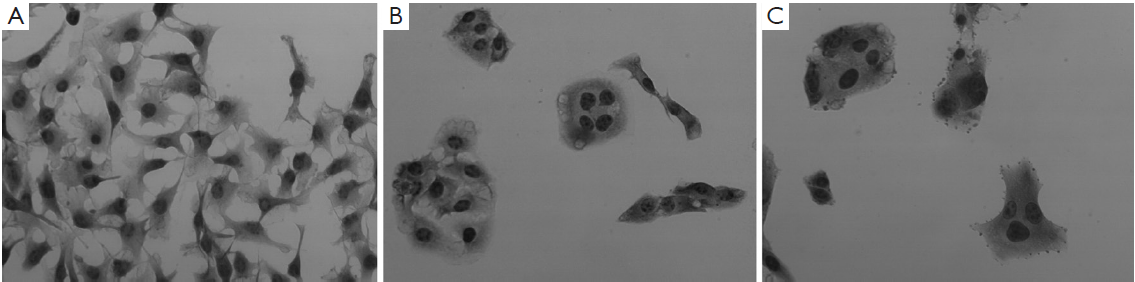
The expression of syncytin-EGFP fusion protein also influenced the invasion potential of B16F10 cells, as determined by scratch wound-healing assay and Matrigel assay. A wound was inflicted into both the B16F10/EGFP and B16F10/Syncytin cell monolayers with 80-90% confluence. After 24 h, B16F10/EGFP cells migrated across and healed the wound, whereas B16F10/Syncytin cells did not (Figure 7A,B). In the Matrigel assays, a large number of B16F10/EGFP cells migrated across the Matrigel layer and appeared on the bottom surface whereas little B16F10/Syncytin cells migrated to the bottom surface (Figure 7C,D). These results indicated that the expression of syncytin decreased the invasion potential of B16F10 melanoma cells.
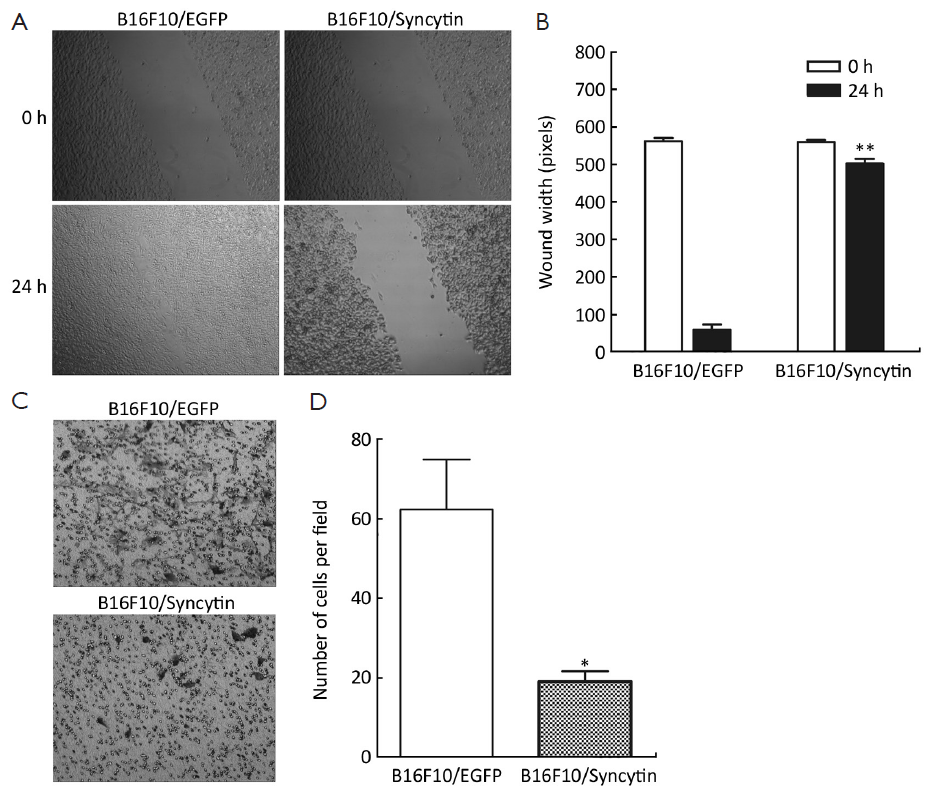
Sensitivity to trichosanthin
Trichosanthin, a ribosome-inactivating protein isolated from the root tubers of Trichosanthes kirilowii Maxim, has been used to induce mid-term abortion and to treat choriocarcinomas (23). Previous studies have shown that syncytiotrophoblast cells, which highly express syncytin protein, are more sensitive to trichosanthin than other cells (23). Thus, we were interested in whether transcribed syncytin in B16F10 cells increased their sensitivity to trichosanthin treatment. The results showed that there was no significant difference in the cell viability between B16F10/EGFP and B16F10/Syncytin cells in the presence of various concentrations of trichosanthin (Figure 8), suggesting that syncytin might not be involved in the action of trichosanthin.
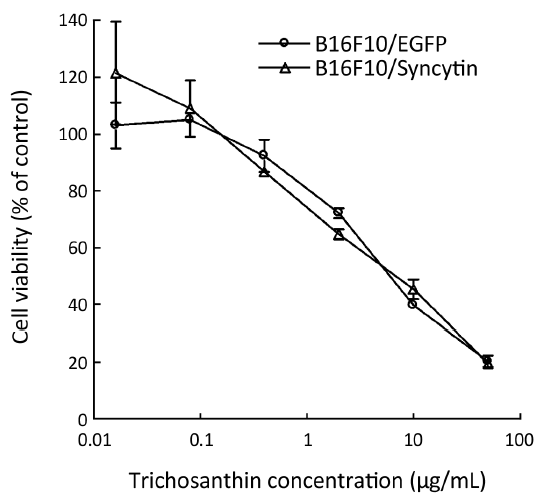
Discussion
The finding of syncytin expression in some tumors implicates its potential values in the prognosis of cancers (16,19,20), since it is not expressed in normal tissues except placenta, testis, brain and osteoclasts (8-10). For example, the protein has been reported to be expressed in multiple sclerosis and leukemia, as well as in breast cancers (18,19,24,25). Yet it is still controversial whether syncytin expression represents positive or negative prognosis (18,20). In this study, we examined the impact of syncytin expression on the phenotypic and invasive properties of tumor cells using a subline of syncytin-expressing B16F10 cells as a model. Our results indicate that syncytin expression have changed the phenotype and significantly confined the proliferation and invasion of B16F10 melanoma cells in in vitro assays, suggesting that the expression of syncytin in some cancers may be a positive prognostic factor.
Syncytin is well-known for its fusogenic activity and plays a key role in cytotrophoblast fusion into syncytiotrophoblasts in human placenta (6,13). It is believed that cell fusion results from the interaction between syncytin and its receptors, such as ASCT-1 and ASCT-2 (3,11,12,14). These receptors are responsible for neutral amino acid transport across cell membranes and are universally expressed in normal tissues. Except for cytotrophoblast fusion, syncytin is involved in cell fusion between tumor and endothelial cells (15) and mediates human osteoclast cell fusion (10). It has been demonstrated that syncytin expression leads to the formation of multinucleated cells (3,15). In line with this observation, our results showed that multinucleated cells were formed in the syncytin-expressing B16F10 cells. Although we cannot currently exclude the possibility of senescence-induced multinucleated cells due to lack of evidence, multinucleation seems likely a result of cell-cell fusion via syncytin and its receptors on the cell surfaces rather than a consequence of karyokinesis without cytokinesis, because some multinucleated cells had only three or five nuclei. In addition, syncytin was tagged with EGFP at its carboxyl terminal as a fusion protein in this study, yet its fusogenic function appeared not affected by this tag since multinucleated cells were induced in the presence of syncytin-EGFP. As it is well known that multinucleated cells usually represents terminally differentiated/aged cells with less proliferation potential, our data suggest that the fusogenic property of syncytin may be involved in the reduction of cell proliferation in syncytin-expressing melanoma cells. Furthermore it has been reported recently that an increase of FasL/Fas expression and cell apoptosis is induced by cyclic AMP in BeWo cells, which coincides with the induction of syncytin and the formation of syncytia (10). Taken together, these results suggest that syncytin expression could restrict the proliferation in some cancers.
In addition to mediating cell-cell fusion, the interaction between syncytin and its receptors is supposed to reduce the migration and invasion of tumor cells. Supporting this, syncytin expression has been reported to serves as a positive prognostic indicator in breast cancers (20). Furthermore, we showed in this study that the highly invasive and metastatic phenotype of B16F10 melanoma cells (22,26) had been converted to low-invasive one which grew tightly together, when they were transfected with a syncytin gene. These results indicate that the fusogenic syncytin is involved in the reduction of the invasion potential of tumor cells.
Syncytin is highly expressed in the placenta especially at the interface of trophoblast and syncytiotrophblasts that is required for normal physiological function of placenta (27,28). Previous studies have indicated that syncytiotrophoblast cells are the most sensitive cells to trichosanthin (23). It is unknown whether syncytin expression renders syncytiotrophoblasts sensitive to trichosanthin. Our results showed that there was no difference in the viabilities of B16F10/Syncytin and B16F10/EGFP cells when exposed to trichosanthin, suggesting that syncytin expression may not have been involved in the sensitivity of syncytiotrophoblasts or choriocarcinoma cells to trichosanthin. Therefore, other mechanisms may be involved in the specific action of trichosanthin on syncytiotrophoblasts.
In conclusion, our results suggest that the fusogenic syncytin may play an important role in restricting the invasiveness of tumor cells. Therefore, syncytin expression represents a positive prognostic factor in patients with some cancers.
Acknowledgements
This work was supported by grants from the Major State Basic Research Development Program of China (973 Program) (No. 2010CB833603) and the National Natural Science Foundation of China (No. 81173604).
Disclosure: The authors declare no conflict of interest.
References
- Malassiné A, Handschuh K, Tsatsaris V, et al. Expression of HERV-W Env glycoprotein (syncytin) in the extravillous trophoblast of first trimester human placenta. Placenta 2005;26:556-62. [PubMed]
- Blond JL, Besème F, Duret L, et al. Molecular characterization and placental expression of HERV-W, a new human endogenous retrovirus family. J Virol 1999;73:1175-85. [PubMed]
- Blond JL, Lavillette D, Cheynet V, et al. An envelope glycoprotein of the human endogenous retrovirus HERV-W is expressed in the human placenta and fuses cells expressing the type D mammalian retrovirus receptor. J Virol 2000;74:3321-9. [PubMed]
- Mangeney M, Renard M, Schlecht-Louf G, et al. Placental syncytins: Genetic disjunction between the fusogenic and immunosuppressive activity of retroviral envelope proteins. Proc Natl Acad Sci U S A 2007;104:20534-9. [PubMed]
- Pötgens AJ, Drewlo S, Kokozidou M, et al. Syncytin: the major regulator of trophoblast fusion? Recent developments and hypotheses on its action. Hum Reprod Update 2004;10:487-96. [PubMed]
- Mallet F, Bouton O, Prudhomme S, et al. The endogenous retroviral locus ERVWE1 is a bona fide gene involved in hominoid placental physiology. Proc Natl Acad Sci U S A 2004;101:1731-6. [PubMed]
- Bonnaud B, Beliaeff J, Bouton O, et al. Natural history of the ERVWE1 endogenous retroviral locus. Retrovirology 2005;2:57. [PubMed]
- Kim HS, Ahn K, Kim DS. Quantitative expression of the HERV-W env gene in human tissues. Arch Virol 2008;153:1587-91. [PubMed]
- Frank O, Giehl M, Zheng C, et al. Human endogenous retrovirus expression profiles in samples from brains of patients with schizophrenia and bipolar disorders. J Virol 2005;79:10890-901. [PubMed]
- Søe K, Andersen TL, Hobolt-Pedersen AS, et al. Involvement of human endogenous retroviral syncytin-1 in human osteoclast fusion. Bone 2011;48:837-46. [PubMed]
- Esnault C, Priet S, Ribet D, et al. A placenta-specific receptor for the fusogenic, endogenous retrovirus-derived, human syncytin-2. Proc Natl Acad Sci U S A 2008;105:17532-7. [PubMed]
- Vargas A, Moreau J, Landry S, et al. Syncytin-2 plays an important role in the fusion of human trophoblast cells. J Mol Biol 2009;392:301-18. [PubMed]
- Huppertz B, Bartz C, Kokozidou M. Trophoblast fusion: fusogenic proteins, syncytins and ADAMs, and other prerequisites for syncytial fusion. Micron 2006;37:509-17. [PubMed]
- Cheynet V, Oriol G, Mallet F. Identification of the hASCT2-binding domain of the Env ERVWE1/syncytin-1 fusogenic glycoprotein. Retrovirology 2006;3:41. [PubMed]
- Bjerregaard B, Holck S, Christensen IJ, et al. Syncytin is involved in breast cancer-endothelial cell fusions. Cell Mol Life Sci 2006;63:1906-11. [PubMed]
- Lin EH, Salon C, Brambilla E, et al. Fusogenic membrane glycoproteins induce syncytia formation and death in vitro and in vivo: a potential therapy agent for lung cancer. Cancer Gene Ther 2010;17:256-65. [PubMed]
- Strick R, Ackermann S, Langbein M, et al. Proliferation and cell-cell fusion of endometrial carcinoma are induced by the human endogenous retroviral Syncytin-1 and regulated by TGF-beta. J Mol Med (Berl) 2007;85:23-38. [PubMed]
- Larsen JM, Christensen IJ, Nielsen HJ, et al. Syncytin immunoreactivity in colorectal cancer: potential prognostic impact. Cancer Lett 2009;280:44-9. [PubMed]
- Sun Y, Ouyang DY, Pang W, et al. Expression of syncytin in leukemia and lymphoma cells. Leuk Res 2010;34:1195-202. [PubMed]
- Larsson LI, Holck S, Christensen IJ. Prognostic role of syncytin expression in breast cancer. Hum Pathol 2007;38:726-31. [PubMed]
- Ouyang DY, Ji YH, Saltis M, et al. Valproic acid synergistically enhances the cytotoxicity of gossypol in DU145 prostate cancer cells: an iTRTAQ-based quantitative proteomic analysis. J Proteomics 2011;74:2180-93. [PubMed]
- Zhang Y, Ouyang D, Xu L, et al. Cucurbitacin B induces rapid depletion of the G-actin pool through reactive oxygen species-dependent actin aggregation in melanoma cells. Acta Biochim Biophys Sin (Shanghai) 2011;43:556-67. [PubMed]
- Shaw PC, Lee KM, Wong KB. Recent advances in trichosanthin, a ribosome-inactivating protein with multiple pharmacological properties. Toxicon 2005;45:683-9. [PubMed]
- Dolei A. MSRV/HERV-W/syncytin and its linkage to multiple sclerosis: the usability and the hazard of a human endogenous retrovirus. J Neurovirol 2005;11:232-5. [PubMed]
- Antony JM, Zhu Y, Izad M, et al. Comparative expression of human endogenous retrovirus-W genes in multiple sclerosis. AIDS Res Hum Retroviruses 2007;23:1251-6. [PubMed]
- Khan ST, Pixley RA, Liu Y, et al. Inhibition of metastasis of syngeneic murine melanoma in vivo and vasculogenesis in vitro by monoclonal antibody C11C1 targeted to domain 5 of high molecular weight kininogen. Cancer Immunol Immunother 2010;59:1885-93. [PubMed]
- Noorali S, Rotar IC, Lewis C, et al. Role of HERV-W syncytin-1 in placentation and maintenance of human pregnancy. Appl Immunohistochem Mol Morphol 2009;17:319-28. [PubMed]
- Dupressoir A, Vernochet C, Harper F, et al. A pair of co-opted retroviral envelope syncytin genes is required for formation of the two-layered murine placental syncytiotrophoblast. Proc Natl Acad Sci U S A 2011;108:E1164-73. [PubMed]