Recombinant adenovirus-p53 (Gendicine) sensitizes a pancreatic carcinoma cell line to radiation
Introduction
In 2012, approximately 44,000 new cases of pancreatic cancer were diagnosed in the United States, making it the tenth most common cancer in both men and women (1). Pancreatic cancer is a lethal disease, with a 5-year relative survival rate for all stages of only 6%. While surgery offers the best chance at prolonged survival, only 10-20% of patients present with resectable disease at the time of diagnosis (2). Even still, locoregional failure remains common in resected cases, and radiation therapy has been used in the neoadjuvant and adjuvant settings to improve local control (3). While the recently published Eastern Cooperative Oncology Group trial showed a survival benefit with the addition of radiation therapy to gemcitabine for locally advanced pancreatic cancer (4), local control at conventional doses remains poor (5). Gemcitabine and fluoropyrimidine-based radiosensitizers are currently in clinical use, but both are associated with moderate to severe toxicities in most patients (4,6). This highlights the urgent need for novel radiosensitizers with less toxicity.
P53, a key mediator of DNA damage and apoptotic responses, is mutated in approximately 60% of pancreatic cancers. Mohiuddin et al. (7) have shown that pancreatic cancer cells with wild-type P53 are significantly more radiosensitive than P53 mutant cells. Another study showed that adenovirus-mediated transfer of wild-type P16 and P53 to pancreatic cancer cells results in multiple effects, including G1 cell cycle arrest and increased apoptosis (8). Recombinant adenovirus-p53 (rAd-p53) (Gendicine; China Shenzhen SiBiono GeneTech Co., Ltd., Shenzhen, China) is a newly developed medicine of gene therapy that relies on the function of wild-type P53; it was recently licenced for clinical use in China for head and neck malignancies. A randomized trial in nasopharyngeal carcinoma has demonstrated improved locoregional control with weekly intratumoral injections of rAd-p53 combined with radiation therapy compared to radiation therapy alone (9). In pancreatic cancer, a pilot study has also demonstrated improved disease control when rAd-p53 is added to chemoradiation therapy (10); however, the mechanism of this combined action is unclear. In the present study, we tested the hypothesis that transfection of pancreatic carcinoma cell line SW1990 (harboring mutant P53) with rAd-p53 can alter expression of target genes, including Bax and p21, and increase sensitivity to radiation. This would provide a scientific foundation for further clinical trials of rAd-p53 as a novel radiosensitizer of pancreatic cancer.
Materials and methods
rAd-p53 and cell culture
rAd-p53 is a recombinant replication-incompetent human serotype 5 adenovirus, in which the E1 region is replaced by a human wild-type p53 expression cassette. rAd-p53 was stored at –20 °C in a concentration of 1×1012 virus particles/mL. The human pancreatic carcinoma cell line SW1990 (mutant p53) was obtained from the Chinese Academy Of Medical Sciences & Peking Union Medical College (CAMS & PUMC, Beijing, China). Cells were cultured in Dulbecco’s modified Eagle’s medium (DMEM) supplemented with 10% fetal bovine serum (FBS; Invitrogen Corporation, Australia), 50 unit/mL penicillin, and 50 µg/mL streptomycin (Invitrogen Corporation, USA) at 37 °C in an atmosphere of 5% CO2 (300/3,000 Incubator, Revco Scientific, USA).
Immunocytochemistry (ICC)
Glass slides were sterilized by dipping them in 90% ethanol and carefully drying them over a flame for a few seconds. Each slide was placed in a sterile 100-mm diameter tissue culture dish, and a specific number of cells were seeded (Table 1). Dishes were divided into an experimental group (group A) and a control group (group B). Cells in group A were infected with rAd-p53 solution at a viral multiplicity of infection (MOI) of 100 (1:100 MOI) for various lengths of time (Table 1). Cells were fixed by incubating them in 4% (V/V) paraformaldehyde in PBS for 20 min at room temperature. Immunocytochemical staining for P53, P21 and Bax was performed using anti-P53 (DO-1; 1/100 dilution; Santa Cruz Biotechnology, USA), anti-P21 (F-5; 1/100 dilution; Santa Cruz Biotechnology, USA), and anti-Bax (B-9; 1/100 dilution; Santa Cruz Biotechnology, USA) antibodies, respectively. ICC-positive cells were stained brown, and ICC-negative cells were stained blue. Slides were visualized using an inverted microscope (Olympus CKX31, Japan). The experiment was repeated three times to minimize random error.

Full table
Cell irradiation
A set number of logarithmic phase cells were grown in 25 cm2 culture flasks, as shown in Table 2. Cells in group A were infected with rAd-p53 solution at a viral MOI of 100. Group B served as the control group. Cultures were terminated by Giemsa staining at different times. The experiment was repeated three times.

Full table
Cells were irradiated with 6 MV X-ray (Varian 600CD linear accelerator, Varian Medical Systems, Inc. USA) at room temperature at a central dose rate of 300 MU/min. The output factor was 1.041, with a 20 cm × 20 cm field. Five clinically relevant doses were chosen: 0 Gy (group 1), 2 Gy (group 2), 4 Gy (group 3), 6 Gy (group 4), and 8 Gy (group 5) (Table 2). Cells were cultured in the CO2 incubator for two weeks and then colony-forming units (≥50 cells) were calculated.
Curve fitting models
Plating efficacy (PE) was defined as colonies observed/number of cells plated. Survival fraction (SF) was the ratio of PE for the irradiation group compared to PE for the control group. Curve fitting analyses were made according to the single-hit multitarget (SHMT) [SF=1–(1–e–D/D0)N, Dq=D0×ln(N)], the modified single-hit multitarget (mSHMT) {S=e–D/D1[1–(1–e–D/D2)]N}, and the linear-quadratic (L-Q) (S=e–αD-βD2) models, which are the most commonly used models in radiation oncology. The mean values were recorded as data.
Statistical analysis
Statistical comparisons were performed using the Pearson chi-square test. A two-tailed P<0.05 was considered statistically significant. SPSS 18.0 (SPSS Inc., Chicago, IL, USA) was used for the calculation of SF prediction, and Origin 8.6 (OriginLab, USA) was used for cell survival curve fitting.
Results
Here, we investigated whether transfection of the pancreatic carcinoma cell line SW1990 with rAd-p53 sensitizes the cells to radiation. Results from ICC staining (Table 3) showed that expressions of P53, P21 and Bax were all significantly increased (P<0.05) after transfection with rAd-p53. Additionally, this increased expression was dependent on time, with the group at 96 h achieving the highest level of expression (Figure 1). Thus, we were able to successfully transfect SW1990 cells with rAd-p53, and this subsequently influenced expression of downstream targets. Average numbers of counted colonies and plating efficiencies are shown in Table 4.
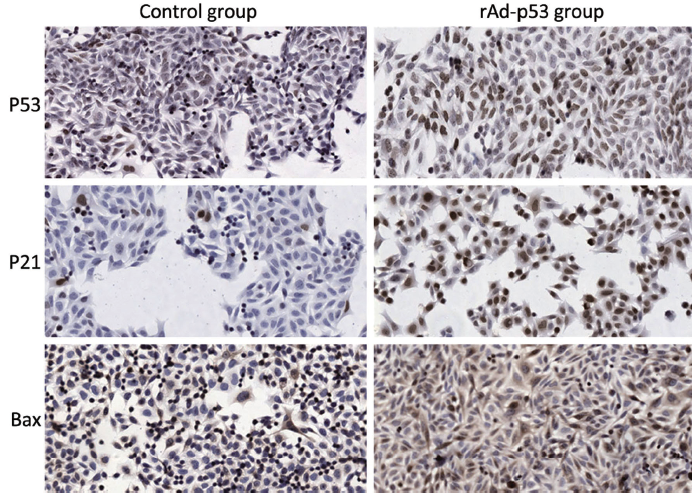
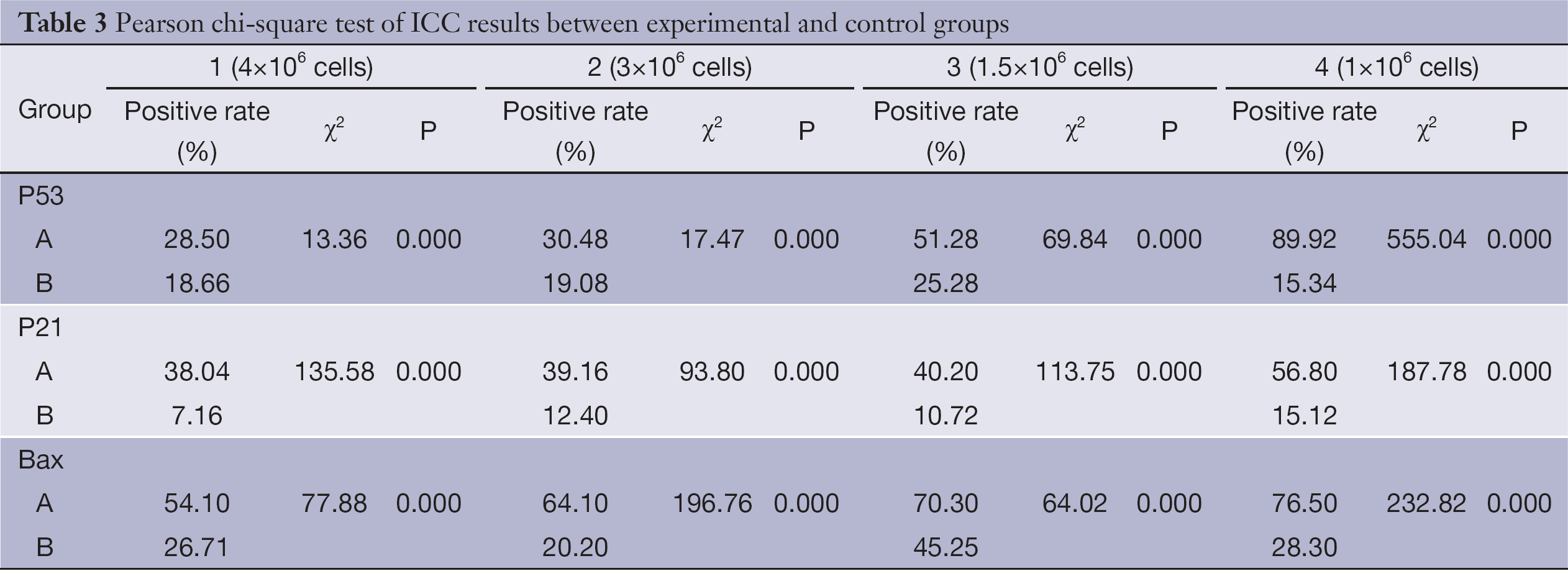
Full table

Full table
We calculated SF of SW1990 cells in both group A and group B at different radiation doses (0, 2, 4, 6 and 8 Gy), and cell survival curves based on the three different models (SHMT, mSHMT, L-Q) were generated (Table 5). Compared to the SHMT and mSHMT models, the L-Q model appeared to give a better fit. Figure 2 demonstrates the prediction of SF with the three different models. Cell survival curves were plotted, and the curve for group A (rAd-p53 infected) was consistently shifted to the left compared to the curve for group B, which suggested that transfection of rAd-p53 enhances the radiosensitivity of SW1990 cells.
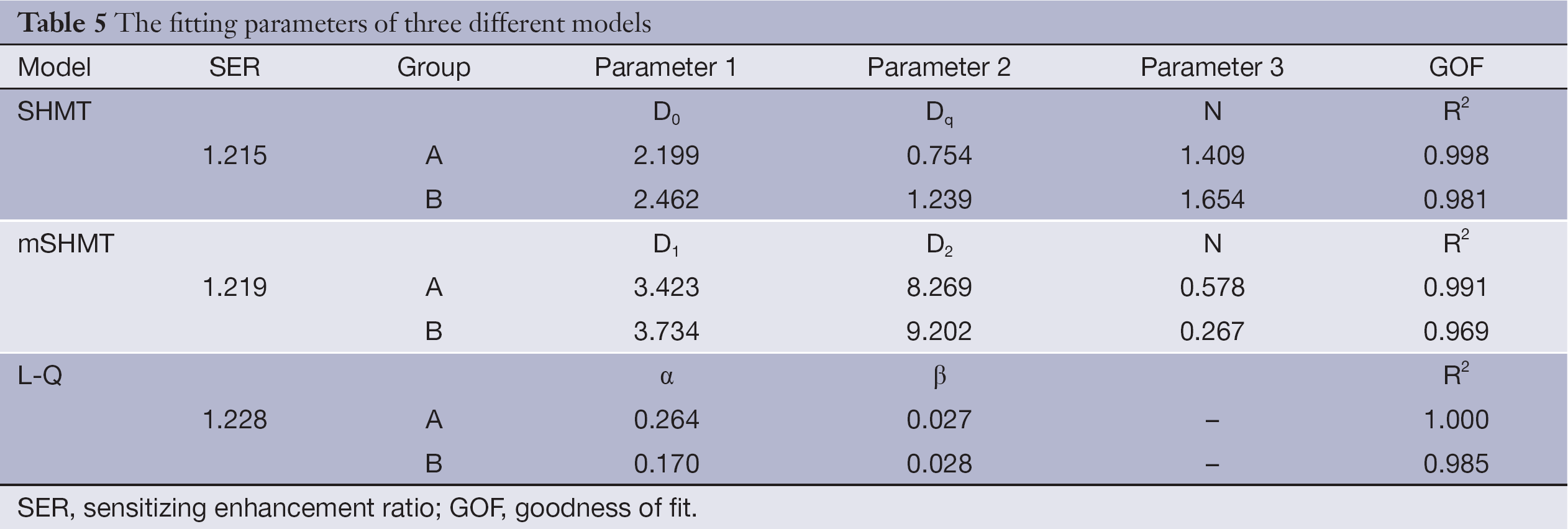
Full table
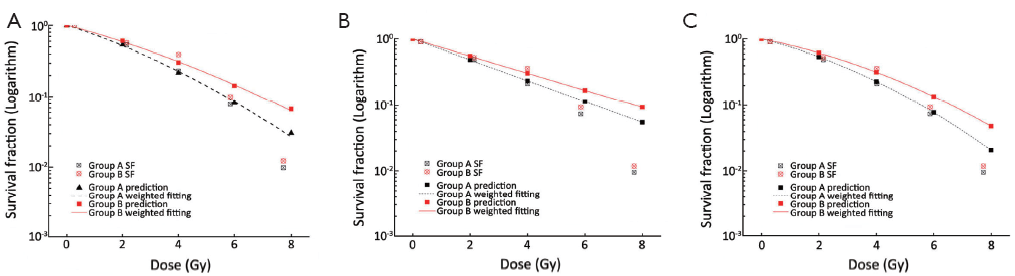
Discussion
Pancreatic cancer is associated with a relatively poor prognosis, prompting research and clinical trials for new and improved therapies (11-14). Gene therapy has emerged as a promising treatment modality in oncology, with more than one thousand clinical trials being conducted worldwide (15). Previous researches on rAd-p53 had been published for esophageal squamous cell carcinoma (16), non-small cell lung cancer (17), ovarian cancer (18), hepatocellular cancer (19), and nasopharygeal cancer (9). However, the applicability and mechanism of action of gene therapy in pancreatic cancer has not been fully elucidated. In this study, we sought to evaluate the effect of rAd-p53 in a pancreatic cancer cell line with mutant p53. We succesfully achieved adenovirus-mediated expression of p53 in SW1990 cells and showed that this expression of wild-type P53 sensitizes the cells to clinically relevant doses of radiation.
We found that rAd-p53 was capable of infecting SW1990 cells, which was similar to previous reports for lung adenocarcinoma cells (20). The infected group showed increased expression of both P21 and Bax compared to the control group. P21 is tightly controlled by P53 and plays a crucial role in mediating growth arrest when cells are exposed to DNA damaging agents, such as radiation. The expression of Bax is upregulated by P53, and Bax has been shown to be involved in P53-mediated apoptosis. The increase in P53 protein expression was time-dependent, with the group at 96 h achieving the highest level of expression. This is in contrast to an earlier study using A549 lung adenocarcinoma cells, which showed no significant difference in P53 expression when comparing the 6 h group to the 24 h group (20). Such difference may be due to the different cancer cell lines used. Further studies need to be performed to identify the peak of P53 expression following infection of this pancreatic cancer cell line.
We found that transfection of SW1990 cells with wild-type p53 increases the radiosensitivity of this cell line, and the L-Q model (sensitizing enhancement ratio, 1.228) gives the best fit for the SF data (Table 5). Our results of successful transfection are similar to the results published by Merlin et al. (21), who used a non-viral technique, and Mercadé et al. (22), who used a viral technique with pro-drug activating enzymes.
This work adds to the body of literature exploring gene therapy in pancreatic cancer. There are still several important questions that must be answered before gene therapy can become a standard treatment option. Adenoviral-mediated expression of P53 (rAd-p53) is currently licensed for clinical use in China. To date, it is unclear whether P53 is an optimal target in pancreatic cancer, and whether there are pre-treatment predictive markers for response. The use of an adenovirus as a delivery system may also pose certain problems. Patients may require multiple intratumoral injections of the virus, as has been previously shown for oral cancer (23). This is primarily die to the fact that the efficacy of expression is quite low when administered intravenously. Additionally, there are safety concerns related to the host immune response (24). However, to date, rAd-p53 has been shown to be associated with only a relatively low toxicity profile (fever) in an earlier trial (10). The experiment should also be performed on other pancreatic cancer cell lines.
In conclusion, the results support the development of gene replacement therapy for pancreatic cancer with rAd-p53 and provide an important preclinical foundation for further work.
Acknowledgements
This study was supported by Fujian Province Natural Science Foundation (No. 2012J05139) and Beijing Municipal Science & Technology Commission (No. Z111107058811021).
Disclosure: The authors declare no conflict of interest.
References
- Siegel R, Naishadham D, Jemal A. Cancer statistics, 2012. CA Cancer J Clin 2012;62:10-29. [PubMed]
- Loos M, Kleeff J, Friess H, et al. Surgical treatment of pancreatic cancer. Ann N Y Acad Sci 2008;1138:169-80. [PubMed]
- Wang F, Kumar P. The role of radiotherapy in management of pancreatic cancer. J Gastrointest Oncol 2011;2:157-67. [PubMed]
- Loehrer PJ Sr, Feng Y, Cardenes H, et al. Gemcitabine alone versus gemcitabine plus radiotherapy in patients with locally advanced pancreatic cancer: an Eastern Cooperative Oncology Group trial. J Clin Oncol 2011;29:4105-12. [PubMed]
- Huguet F, Goodman KA, Azria D, et al. Radiotherapy technical considerations in the management of locally advanced pancreatic cancer: american-French consensus recommendations. Int J Radiat Oncol Biol Phys 2012;83:1355-64. [PubMed]
- Lombardi L, Troiano M, Silvestris N, et al. Combined modality treatments in pancreatic cancer. Expert Opin Ther Targets 2012;16 Suppl 2:S71-81. [PubMed]
- Mohiuddin M, Chendil D, Dey S, et al. Influence of p53 status on radiation and 5-flourouracil synergy in pancreatic cancer cells. Anticancer Res 2002;22:825-30. [PubMed]
- Ghaneh P, Greenhalf W, Humphreys M, et al. Adenovirus-mediated transfer of p53 and p16(INK4a) results in pancreatic cancer regression in vitro and in vivo. Gene Ther 2001;8:199-208. [PubMed]
- Pan JJ, Zhang SW, Chen CB, et al. Effect of recombinant adenovirus-p53 combined with radiotherapy on long-term prognosis of advanced nasopharyngeal carcinoma. J Clin Oncol 2009;27:799-804. [PubMed]
- Li JL, Cai Y, Zhang SW, et al. Combination of Recombinant Adenovirus-p53 with Radiochemotherapy in Unresectable Pancreatic Carcinoma. Chin J Cancer Res 2011;23:194-200. [PubMed]
- Herman JM, Wild AT, Wang H, et al. Randomized phase III multi-institutional study of TNFerade biologic with fluorouracil and radiotherapy for locally advanced pancreatic cancer: final results. J Clin Oncol 2013;31:886-94. [PubMed]
- Boeck S, Jung A, Laubender RP, et al. EGFR pathway biomarkers in erlotinib-treated patients with advanced pancreatic cancer: translational results from the randomised, crossover phase 3 trial AIO-PK0104. Br J Cancer 2013;108:469-76. [PubMed]
- Duong HQ, Hong YB, Kim JS, et al. Inhibition of checkpoint kinase 2 (CHK2) enhances sensitivity of pancreatic adenocarcinoma cells to gemcitabine. J Cell Mol Med 2013;17:1261-70. [PubMed]
- Wei D, Parsels LA, Karnak D, et al. Inhibition of Protein Phosphatase 2A Radiosensitizes Pancreatic Cancers by Modulating CDC25C/CDK1 and Homologous Recombination Repair. Clin Cancer Res 2013;19:4422-32. [PubMed]
- Curtin JF, Candolfi M, Xiong W, et al. Turning the gene tap off; implications of regulating gene expression for cancer therapeutics. Mol Cancer Ther 2008;7:439-48. [PubMed]
- Shimada H, Matsubara H, Shiratori T, et al. Phase I/II adenoviral p53 gene therapy for chemoradiation resistant advanced esophageal squamous cell carcinoma. Cancer Sci 2006;97:554-61. [PubMed]
- Swisher SG, Roth JA, Komaki R, et al. Induction of p53-regulated genes and tumor regression in lung cancer patients after intratumoral delivery of adenoviral p53 (INGN 201) and radiation therapy. Clin Cancer Res 2003;9:93-101. [PubMed]
- Wen SF, Mahavni V, Quijano E, et al. Assessment of p53 gene transfer and biological activities in a clinical study of adenovirus-p53 gene therapy for recurrent ovarian cancer. Cancer Gene Ther 2003;10:224-38. [PubMed]
- Tu K, Zheng X, Zhou Z, et al. Recombinant human adenovirus-p53 injection induced apoptosis in hepatocellular carcinoma cell lines mediated by p53-Fbxw7 pathway, which controls c-Myc and cyclin E. PloS one 2013;8:e68574. [PubMed]
- Ma JT, Han CB, Zhao JZ, et al. Synergistic cytotoxic effects of recombinant human adenovirus p53 and radiation at various time points in A549 lung adenocarcinoma cells. Oncol Lett 2012;4:529-33. [PubMed]
- Merlin JL, Dolivet G, Dubessy C, et al. Improvement of nonviral p53 gene transfer in human carcinoma cells using glucosylated polyethylenimine derivatives. Cancer Gene Ther 2001;8:203-10. [PubMed]
- Mercadé E, Cascalló M, Carrió M, et al. Treatment based on a combination of the CYP2B1/cyclophosphamide system and p53 delivery enhances tumour regression in human pancreatic cancer. Ann Oncol 2001;12:379-88. [PubMed]
- Liu S, Chen P, Hu M, et al. Randomized, controlled phase II study of post-surgery radiotherapy combined with recombinant adenoviral human p53 gene therapy in treatment of oral cancer. Cancer Gene Ther 2013;20:375-8. [PubMed]
- Patil SD, Rhodes DG, Burgess DJ. DNA-based therapeutics and DNA delivery systems: a comprehensive review. AAPS J 2005;7:E61-77. [PubMed]