The effect of beta-elemene on alpha-tubulin polymerization in human hepatoma HepG2 cells
Hepatocellular carcinoma (HCC) is a severe life threatening disease as the fifth most common cancer worldwide (1,2). It belongings to chemotherapy nonsensive cancer, clinical reports show that the benefits of both chemotherapy and target antitumor drugs for HCC are not satisfactory (3-6). Therefore searching for new effective agents against hepatoma is anxiously expected.
Beta-elemene is a non-cytotoxic antitumor drug, which is extracted from a traditional Chinese medical herb Rhizoma zedoariae. It has been clinical used in china for decades. Clinical data shows it can effectively inhibit the growth of various tumors without severe side effects, especially for HCC (7-10). Studies show that beta-elemene can kill tumor cells by inducing apoptosis of tumor cells, blocking the cell cycle and inhibiting signal transduction (10-16). However, the relationship between beta-elemene and microtuble is rarely reported.
Microtubule, polymerized by alpha–tubulin and beta-tubulin, is an important component of the cytoskeleton. It plays an important role in cell growth, maintenance of cell shape, cell signal transduction and mitotic processes ect., and acts as an essential tumor target. The important anti-tumor drugs, such as paclitaxel, docetaxel, and vincristine, inhibit cancer growth by promoting the over-dimerization or over-destabilization of microtubule in the cells (17,18).
This study is designed to investigate the impact of beta-elemene injection on the growth and alpha-tubulin of human hepatocarcinoma HepG2 cells, and at the same time discussed the inhibiting mechanism of beta-elemene injection on HCC.
Materials and methods
Agents and implements
Beta-elemene injection (Dalian Jingang Pharmaceutical Corporation, China, more than 99%, #0508031), MTT (Biosharp Company, USA), dimethyl sulfoxide (DMSO) (Amersco), RT-PCR kit (TaKaPa Company), alpha-tubulin mouse anti-human monoclonal antibody (Biyuntian Biotechnology Research Institute), horseradish peroxidase-labeled Goat anti-Mouse IgG (Beijing Zhongshan Jinqiao biological Corporation), PVDF membrane.
Cell lines and culture conditions
Human hepatoma HepG-2 cells (Cell Bank of Chinese Academy of Sciences, Shanghai, China) were cultured with IMDM medium, supplemented with 10% (v/v) fetal calf serum (FCS). The cultures were maintained at 37 °C in a humidified atmosphere of 5% CO2.
MTT assay for cell proliferation
5,000 cells/well were seeded in 96-well plates. After 24 h of incubation, the treated group were added to beta-elemene injection with different concentrations (0.1, 0.08, 0.06, 0.04, 0.02, 0.01 mg/mL perspectively). After incubation for 24, 48, 72 h, 20 µL of MTT dye solution (5 g/mL, in phosphate buffer pH 7.4) was added to each well and incubated at 37 °C for 4 h. The medium was removed and formazan was dissolved in 150 µL of DMSO and the absorbance (A) at 570 nm of each group was measured by enzyme-labeled instrument. Results were expressed as the mean percentage of tumor growth inhibition rate (IR) and half inhibition concentration (IC50). IR% = (Acontrol – Atreated)/(Acontrol – Ablank) ×100%. The experiments were repeated three times.
Flow cytometry (FCM) analysis for tumor cell cycle distribution
A total of 2×106 HepG-2 cells were seeded and added to beta-elemene injection with different concentrations (0.02, 0.04, 0.08 mg/mL) for 24 h. Then they were harvested, washed and fixed in 70% ice cold ethanol at 4 °C overnight. Afterwards, cells were washed twice and resuspended in 1 mL of propidium iodide (PI) stain solution (l µg/mL PI, 1% TritonX-100 in PBS) containing 2 mg/mL RNase A at 37 °C in the dark for 30 min. The cells were analyzed and fluorescence detected immediately by FCM of their apoptosis and cell cycle (excitation wave 562 nm, emission wave 630 nm). The experiments were repeated three times.
RT-PCR detection of alpha-tubulin mRNA expression
Cells in the exponential growth phase (5×105 cells/well) were seeded in 24-well plates, and cultured for 24 h. Beta-elemene injection with different concentrations(0.02, 0.04, 0.08 mg/mL) were added to the treated group. After cultured for 24 h, the cells were collected. Total RNA was isolated from cells using Trizol (Invitrogen Company, USA). RT-PCR was performed following the instruction of TaKaRa RNA PCR Kit (AMV) Ver3.0. Primer pairs used for alpha-tubulin (158 bp) were 5'-ATGGAGCCCTGAATGTTGAC-3', 5'-CTCAAAGCAAGCATTGGTGA-3'. As to GAPDH(226 bp), its Primer pairs were 5'-GAAGGTGAAGGTCGGAGT-3', 5'-GAAGATGGTGATGGGATTTC-3', PCR reaction conditions were as follows: initial denaturation temperature of 95 °C for 5 min, denaturation temperature of 95 °C for 30 s, annealing temperature of 60 °C 30 s, extension temperature of 72 °C for 30 s (cycling 30 times).
The amplified products were analyzed by agarose gel electrophoresis using 1% gel with ethidium bromide staining. The bands were analyzed with Jim-250. The experiments were repeated three times.
Western Blot analysis for alpha-tubulin protein expression
HepG-2 cells were treated with different concentrations of beta-elemene injection (0.02, 0.04, 0.08 mg/mL) for 24 h before they were collected and washed. Then the treated groups were added with 200 µL lysis buffer [1.5 M Tris-HCl (pH 6.8) 41.5 µL/L, 20% SDS 100 µL/L, glycerine 100 µL/L, dithiothreitol 0.0155 g/L, bromophenol blue 0.000125 g/L] on the ice for 10 s. After collected and boiled in water at 100 °C for 7 min, the content of the total protein was determined using the method of Bradford. Equal amounts of protein (50 µg/hole) were subjected to SDS-PAGE on 8-12% separation gel for electrophoresis. Afterwards, the proteins were electrophoretically transferred to PVDF membranes, blocked at room temperature for 1 h, and then treated with alpha-tubulin mouse anti-Human monoclonal antibody overnight. After washed by PBS/T, the membranes were incubated in horseradish peroxidase-labeled goat antimouse IgG (1:5000) at room temperature for 1h, and then treated with the ECL reagent. Finally, X-ray films were exposed for 1-5 min, developed, dried, scanned and photographed. The experiments were repeated three times.
Detection of polymerized microtubules
The proportion of polymerized of microtubules was detected based on the protocol as previously described (19). HepG-2 cells were treated with different doses of beta-elemene injection (0.02, 0.04, 0.08 mg/mL) for 24 h before they were collected and washed. Then the treated groups were added with 200 µL hypotonic lysis buffer (MgCl2 1 mmol/L, EGTA 2 mmol/L, 0.5% NP40, PMSF 2 mmol/L, 10 µL of protease inhibitor, Tris-HCl (pH 6.8) 20 mmol/L) at 37 °C in dark for 10 min. After centrifuged at 14,000 r/min for 10 min. The supernatant and the precipitate were collected respectively. The supernatant containing soluble tubulin, while the precipitate was resuspended with 100 µL hypotonic buffer, this portion of the supernatant containing the original polymerized tubulin. Both the polymerized tubulin and soluble tubulin were detected at protein level, using Western blot analysis with the former (Considering tubulin protein content decreased because of drug effects, we cautiously increased the amount of protein samples in drug treatment groups).
Statistical analysis
Statistical analysis was performed using SPSS14.0 statistical software. All experimental data are presented as mean ± SD. The data between multiple groups was compared by single-factor analysis of variance, and P<0.05 was considered statistically significant.
Results
Beta-elemene inhibited HepG2 cells proliferation
MTT assay showed that after 24, 48, 72 h interference of beta-elemene injection with different concentrations, IR of beta-elemene injection on HepG2 cells were increased when interference time and drug concentration increased, illustrating time- and dose-dependent ways (Figure 1).
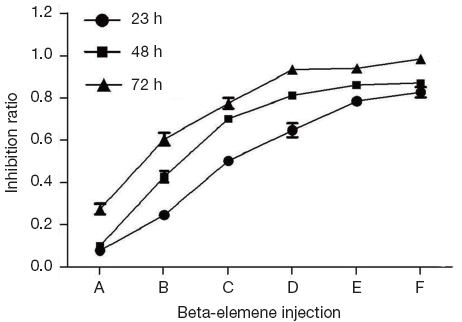
Flow cytometry analysis of tumor cell cycle distribution
After treated by beta-elemene injection with different concentrations (0.02, 0.04, 0.08 mg/mL) for 24 h, HepG-2 cells were analyzed by FCM. The rate of HepG-2 cells at S phase increased when interference dose of beta-elemene injection increased (Figure 2), significantly higher than that of the control group (P<0.05), illustrating a dose-dependent way (Table 1).
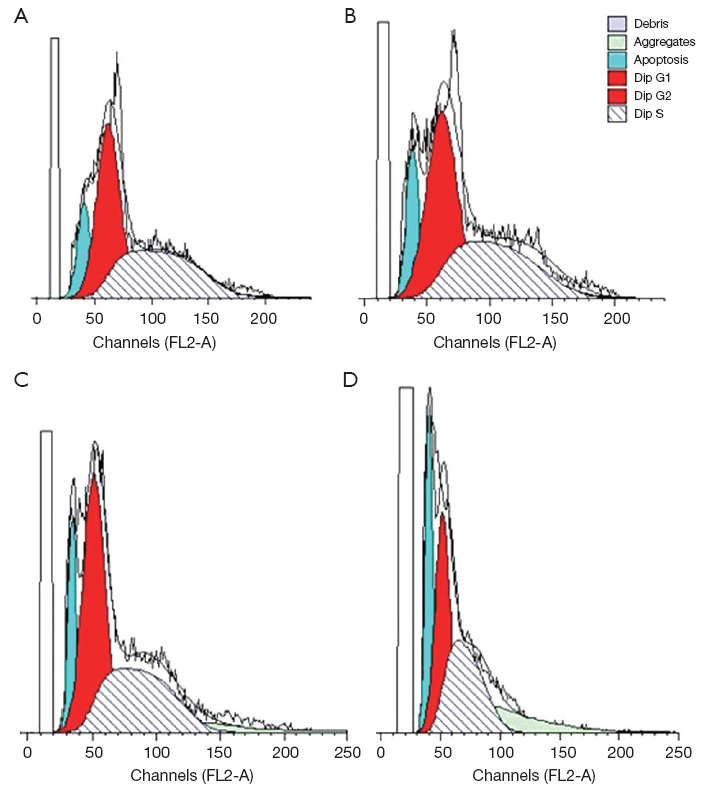

Full table
Detection of alpha-tubulin mRNA expression by RT-PCR
After 24 h interference of beta-elemene injection with different concentrations, the relative mRNA level of alpha-tubulin was determined by RT-PCR. Corresponding to beta-elemene injection with 0.02, 0.04, 0.08 mg/mL respectively, the relative alpha-tubulin mRNA level (alpha-tubulin mRNA/GAPDH mRNA) were 0.746±0.018, 0.456±0.035, 0.274±0.025, significantly lower than that of the control group (0.923±0.009, P<0.05), illustrating a dose-dependent way (Figure 3).
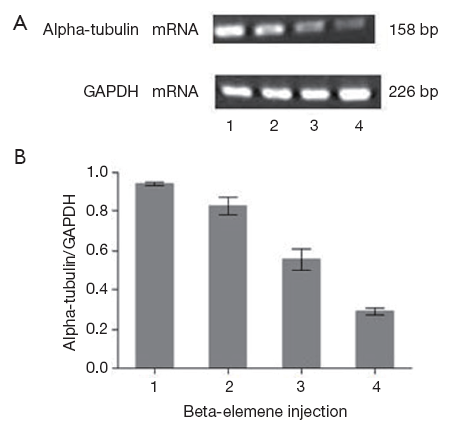
Detection of alpha-tubulin protein expression by Western Blot
After 24 h interference of beta-elemene injection with different concentrations, the relative protein level of alpha-tubulin was detected by Western Blot. Corresponding to beta-elemene injection with 0.02, 0.04, 0.08 mg/mL respectively, the relative alpha-tubulin protein level (alpha-tubulin protein/GAPDH protein) were 0.575±0.006, 0.286±0.014, 0.224±0.015, significantly lower than that of the control group (0.710±0.010, P<0.05), illustrating a dose-dependent way (Figure 4).
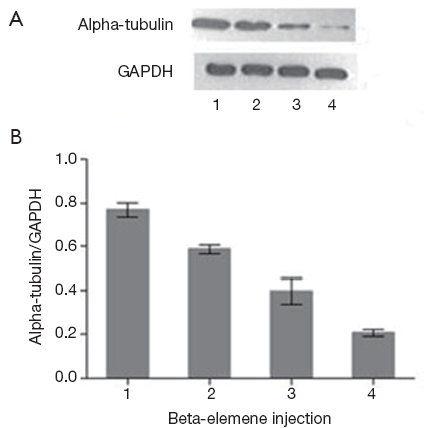
Analysis of polymerized microtubules by Western blot
After 24 h interference of beta-elemene injection with different concentrations, both the relative polymerized tubulin and soluble tubulin were detected at protein level by Western Blot. With the concentration of beta-elemene injection increasing, the relative ratio of polymerized tubulin to total tubulin (polymerized tubulin + soluble tubulin) gradually decreased (In the detection of microtubules, considering tubulin protein content decreased because of drug effects, we cautiously increased the amount of protein samples in drug treatment groups. As a result, it looked like that the total soluble alpha-tubulin protein level did not change too much). The treated group was significantly lower than that of the control group (P<0.05; Figure 5).
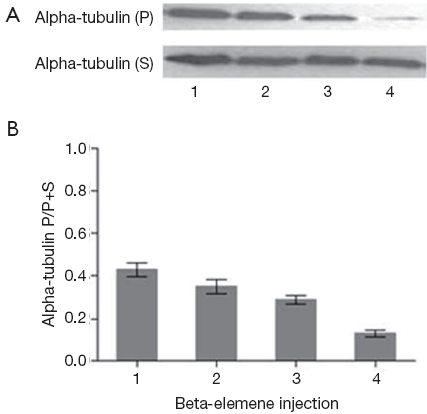
Discussion
Nowadays, drugs for HCC are far below satisfactory. Clinical reports show that the partial response (PR) of hepatoma to chemotherapy is less than 20% up to now (3,4). Also, as the most promising target antitumor drug for HCC, sorafenib only shows approximate three months survival advantage over the untreated group (5,6). Therefore, drugs with new mechanism are anxiously expected in HCC therapy.
Beta-elemene exhibits anticancer effects in various human tumors such as HCC, ovarian cancer and cervical cancer without severe side effects (20-23). No bone marrow suppression has been observed; on the contrary, patient immunity was improved (24). Study show that beta-elemene and cisplatin may be a potential combinatorial therapy to circumvent cisplatin drug resistance in patients with HCC, ovarian cancer and other malignancies (10).
Current studies show that, the antitumor mechanisms of beta-elemene involve apoptosis induce, cell cycle arrest, signal transduction interference, tumor angiogenesis and metastasis inhibition (10-16). However, the impact of beta-elemene on tumor microtubule system is rarely reported.
In this study, we showed that beta-elemene injection can inhibit human hepatoma HepG2 cells growth, induce cell apoptosis, and induce cell cycle arrested at S phase. It can also down-regulated alpha-tubulin at both mRNA and protein levels, presenting a dose-dependent manner.
Microtubule is an essential component of the cytoskeleton. It is involved in multiple processes such as cell growth, maintenance of cell shape, cell signal transduction and mitotic processes. Interfering with microtubule polymerization and decomposition is an important mechanism for tumor drug therapy. Some tumor drugs, such as paclitaxel and vincristine, kill carcinoma cells by Interrupting tubulin synthesis and induce cell cycle arrested at G2/M phase (10,11). There are also compounds, such as cyclic ethyl ester series derivatives, natural products Artelastin and beta-Valley sterol ect., inhibit microtubule assembly and induce cell cycle arrested at S phase (25-28), which is consistent with our conclusion. The researchers have explained the S phase-arrested mechanism of the compounds on tumor as follows: drugs interfere the combination of mitochondria with microtubules, and then interrupt ATP/ADP conversion and energy supply, and further cause tumor cells arrested at S phase. However, the mechanism of beta-elemene injection inducing HCC arrested at S phase needs further research.
In summary, our study suggested beta-elemene injection can inhibit the proliferation of hepatoma HepG2 cells and induce cell apoptosis, the mechanism might be partly related to the down-regulation of alpha-tubulin and inhibition of microtubular polymerization. Beta-elemene is a promising drug on HCC therapy.
Acknowledgements
The General Program of National Natural Science Foundation of China: Research Fund for the mechanism of Arenobufagin space isomer inhibits lymphatic metastasis of mouse hepatocarcinoma. The Project Sponsored by the Scientific Research Foundation for the Returned Overseas Chinese Scholars, State Education Ministry; Specialized Research Fund for the Doctoral Program of Higher Education (NO.20102105120002).
Disclosure: The authors declare no conflict of interest.
References
- McGlynn KA, London WT. The global epidemiology of hepatocellular carcinoma: present and future. Clin Liver Dis 2011;15:223-43. [PubMed]
- Cabrera R, Limaye AR, Horne P, et al. The anti-viral effect of sorafenib in hepatitis C-related hepatocellular carcinoma. Aliment Pharmacol Ther 2013;37:91-7. [PubMed]
- Sun Y, Lu XX, Cui XN, et al. Impact of Cinobufacini injection on proliferation and cell cycle of human hepatoma HepG-2 cells. Chinese-German Journal of Clinical Oncology 2011;10:321-4.
- Sun Y, Shi YK. eds. Clinical tumor medicine manual. 5th ed. Peking: People Health Publisher, 2007;539-49.
- Llovet JM, Ricci S, Mazzaferro V, et al. Sorafenib in advanced hepatocellular carcinoma. N Engl J Med 2008;359:378-90. [PubMed]
- Cheng AL, Kang YK, Chen Z, et al. Efficacy and safety of sorafenib in patients in the Asia-Pacific region with advanced hepatocellular carcinoma: a phase III randomised, double-blind, placebo-controlled trial. Lancet Oncol 2009;10:25-34. [PubMed]
- Yang FQ, Li SP, Chen Y, et al. Identification and quantitation of eleven sesquiterpenes in three species of Curcuma rhizomes by pressurized liquid extraction and gas chromatography-mass spectrometry. J Pharm Biomed Anal 2005;39:552-8. [PubMed]
- Zhou X, Li Z, Liang G, et al. Analysis of volatile components of Curcuma sichuanensis X. X. Chen by gas chromatography-mass spectrometry. J Pharm Biomed Anal 2007;43:440-4. [PubMed]
- Zhang HY, Hu CX, Liu CP, et al. Screening and analysis of bioactive compounds in traditional Chinese medicines using cell extract and gas chromatography-mass spectrometry. J Pharm Biomed Anal 2007;43:151-7. [PubMed]
- Li X, Wang G, Zhao J, et al. Antiproliferative effect of beta-elemene in chemoresistant ovarian carcinoma cells is mediated through arrest of the cell cycle at the G2-M phase. Cell Mol Life Sci 2005;62:894-904. [PubMed]
- Wang G, Li X, Huang F, et al. Antitumor effect of beta-elemene in non-small-cell lung cancer cells is mediated via induction of cell cycle arrest and apoptotic cell death. Cell Mol Life Sci 2005;62:881-93. [PubMed]
- Tao L, Zhou L, Zheng L, et al. Elemene displays anti-cancer ability on laryngeal cancer cells in vitro and in vivo. Cancer Chemother Pharmacol 2006;58:24-34. [PubMed]
- Chen Z, Song Y, Che J, et al. Validation of a sensitive gas chromatographic-mass spectrometric method for the simultaneous determination of beta-elemene and beta-elemenal in human plasma. J Chromatogr B Analyt Technol Biomed Life Sci 2009;877:408-14. [PubMed]
- Peng X, Zhao Y, Liang X, et al. Assessing the quality of RCTs on the effect of beta-elemene, one ingredient of a Chinese herb, against malignant tumors. Contemp Clin Trials 2006;27:70-82. [PubMed]
- Sun Y, Liu G, Zhang Y, et al. Synthesis and in vitro anti-proliferative activity of beta-elemene monosubstituted derivatives in HeLa cells mediated through arrest of cell cycle at the G1 phase. Bioorg Med Chem 2009;17:1118-24. [PubMed]
- Xu L, Tao S, Wang X, et al. The synthesis and anti-proliferative effects of beta-elemene derivatives with mTOR inhibition activity. Bioorg Med Chem 2006;14:5351-6. [PubMed]
- Górka M, Daniewski WM, Gajkowska B, et al. Autophagy is the dominant type of programmed cell death in breast cancer MCF-7 cells exposed to AGS 115 and EFDAC, new sesquiterpene analogs of paclitaxel. Anticancer Drugs 2005;16:777-88. [PubMed]
- Nicholson B, Lloyd GK, Miller BR, et al. NPI-2358 is a tubulin-depolymerizing agent: in-vitro evidence for activity as a tumor vascular-disrupting agent. Anticancer Drugs 2006;17:25-31. [PubMed]
- Kavallaris M, Tait AS, Walsh BJ, et al. Multiple microtubule alterations are associated with Vinca alkaloid resistance in human leukemia cells. Cancer Res 2001;61:5803-9. [PubMed]
- Fu NW. Antitumor effect and pharmacological actions of beta-elemene isolated from the rhizome of Curcuma aromatica. Zhong Yao Tong Bao 1984;9:83-7. [PubMed]
- Tan P, Zhong W, Cai W. Clinical study on treatment of 40 cases of malignant brain tumor by elemene emulsion injection. Zhongguo Zhong Xi Yi Jie He Za Zhi 2000;20:645-8. [PubMed]
- Wang J, Zhang H, Sun Y. Phase III clinical trial of elemenum emulsion in the management of malignant pleural and peritoneal effusions. Zhonghua Zhong Liu Za Zhi 1996;18:464-7. [PubMed]
- Hu J, Jin W, Yang PM. Reversal of resistance to adriamycin in human breast cancer cell line MCF-7/ADM by beta-elemene. Zhonghua Zhong Liu Za Zhi 2004;26:268-70. [PubMed]
- Wu W, Liu K, Tang X. Preliminary study on the antitumor immuno-protective mechanism of beta-elemene. Zhonghua Zhong Liu Za Zhi 1999;21:405-8. [PubMed]
- Wang L, Yang YJ, Chen SH, et al. Effects of beta-sitosterol on microtubular systems in cervical cancer cells. Zhonghua Yi Xue Za Zhi 2006;86:2771-5. [PubMed]
- Davis A, Jiang JD, Middleton KM, et al. Novel suicide ligands of tubulin arrest cancer cells in S-phase. Neoplasia 1999;1:498-507. [PubMed]
- Jiang JD, Denner L, Ling YH, et al. Double blockade of cell cycle at g(1)-s transition and m phase by 3-iodoacetamido benzoyl ethyl ester, a new type of tubulin ligand. Cancer Res 2002;62:6080-8. [PubMed]
- Pedro M, Ferreira MM, Cidade H, et al. Artelastin is a cytotoxic prenylated flavone that disturbs microtubules and interferes with DNA replication in MCF-7 human breast cancer cells. Life Sci 2005;77:293-311. [PubMed]