Circulating tumor cell isolation: the assets of filtration methods with polycarbonate track-etched filters
Introduction
Circulating tumor cells (CTCs) arise from primary or secondary tumors and enter the bloodstream by active or passive intravasation (1). Some of these cells may be responsible for metastasis after gaining properties of extravasation, survival and proliferation in the target tissue. Given their low frequency in the blood, 1 CTC/106-108 hematopoietic cells (2), the identification of CTCs must be preceded by enrichment. The two most common enrichment techniques are filtration method and immuno-magnetic antigen-dependent technique. The latter selects CTCs by expression of membrane proteins. Each technique isolates different subgroups of CTC related to their heterogeneity (3).
Filtration methods are based on physical properties that allow isolation of CTCs by size. Indeed, CTCs are generally larger in size than hematopoietic cells, so most of these cells pass through the filter whereas CTCs are retained. Since the first demonstration of CTC isolation by filtration in 1964 (4), filtration devices have been improved and many downstream applications have been developed. In this review, we present only commercially available methods using polycarbonate track-etched filters. We develop the pros and cons of this technique compared to other enrichment methods. Furthermore, we review applications and the clinical utility of CTC filtration method.
Technique for cytomorphological analysis
Three filtration devices are commercially available (Table 1). For cytology, the most frequently used filtration methods are performed using Screencell®Cyto and ISET® devices, with track-etched polycarbonate filter. The filter pores measure 7.5 to 8 µm in diameter and retain 85-100% of tumor cells and only 0.1% of common blood cells (5).
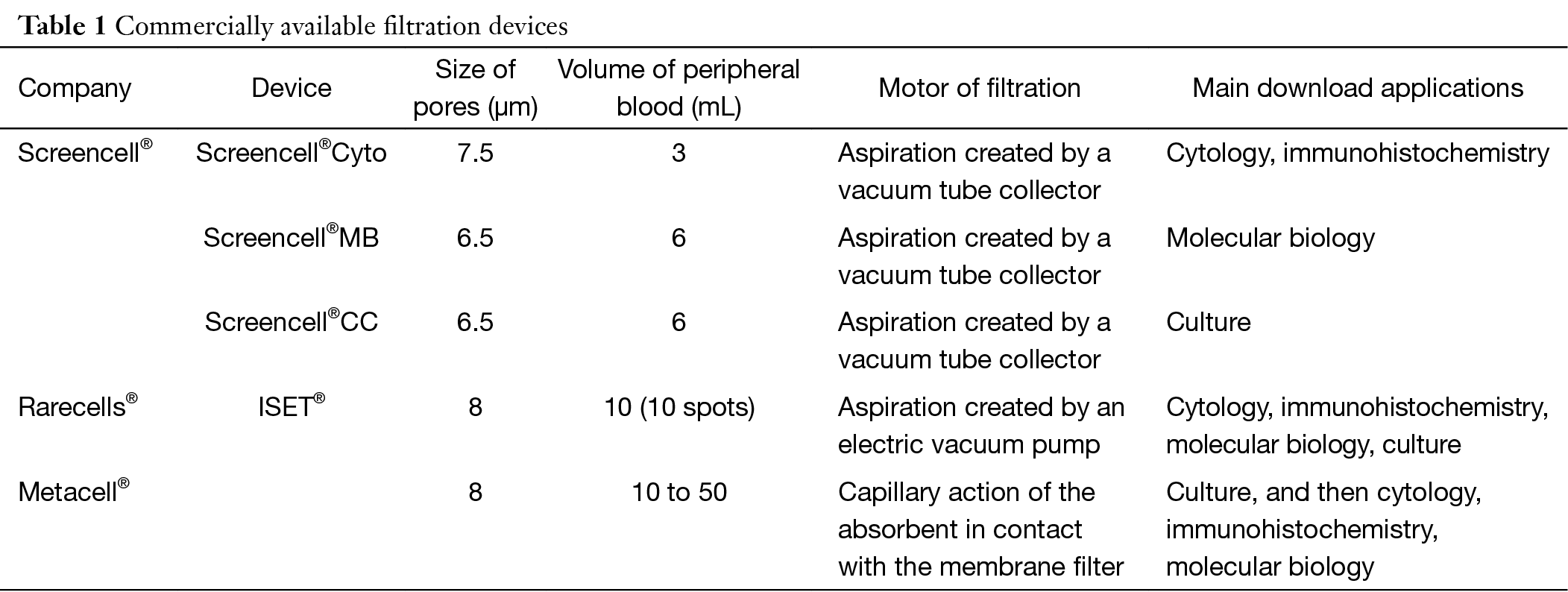
Full table
The blood sample, collected in EDTA tube, is stored at 4 °C maximum 4 hours before filtration.
In Screencell®Cyto device, 3 mL of peripheral blood sample is diluted in 4 mL of filtration buffer mainly composed of saponin and paraformaldehyde (the patented formula is protected) during 8 minutes at room temperature. The 7 mL diluted sample is then filtrated in a unique filtration column by aspiration created by a vacuum tube collector. The device used is disposable (6).
In ISET® device commercialized by Rarecell®, peripheral blood is diluted 1:10 in the filtration buffer (0.175% saponin, 0.2% paraformaldehyde, 0.0372% EDTA, and 0.1% bovine serum albumin) for 10 minutes at room temperature. The module of filtration has 12 wells, enabling filtration of 12 samples of 10 mL of diluted solution. Each well includes a 0.6-cm-diameter surface filter. Filtration is initiated by aspiration created by a vacuum pump (7).
After blood filtration by Screencell®Cyto or RareCell® systems, the filtration membrane is disassembled from the module allowing staining or other techniques.
Hematoxylin-eosin or May-Grunwald-Giemsa stain may be performed on the filters. After staining and glass mounting, morphological analysis is carried out using routine optical microscope. CTC malignancy is identified using morphologic cytopathology criteria.
An alternative size-based separation technique is based on filtration driven by capillary-action (8). Metacell® have develop a commercially device using this technique for CTC culture which allows cytological identification. This device is presented in detail in the culture cell paragraph.
Advantages and disadvantages
CTC isolation by size method is fast, simple and reliable: filtration and coloration is an easy process and cytopathologists can identify CTCs using classic cytopathologic criteria (Figure 1). No complex instruments or adapted staff training is needed, but the help of a trained cytopathologist is mandatory to analyze the stained filters. A technical imperative however is the treatment of the sample within 4 hours of collection. An infrequent technical complication is characterized by blood clogging on the filter which stops filtration.
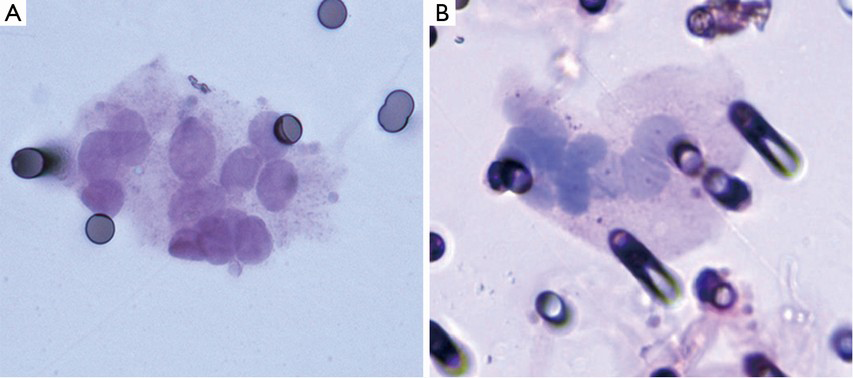
The enrichment device selects tumor cells on size criteria. Conversely, most PCR-based methods, immuno-magnetic techniques such as CellSearch® or other label-dependent microfluidic techniques retain only EpCAM positive cells. It is well know that tumor cells undergo epithelial-mesenchymal transition phenomena during tumorigenesis which enhances the invasive potential of the tumor cell (9). During this process, tumor cells lose the expression of epithelial markers and can acquire a mesenchymal or a stem-cell like phenotype (10). This population of tumor cells, missed by CellSearch® (11), seems to be critical in metastasis initiation (9). Another hypothesis of metastasis initiation is the invasion of target tissue by microemboli (12), which is well analyzed by filtration systems, contrary to CellSearch® (13). Indeed, these oligoclonal clusters reach a 23- to 50-fold increased metastatic potential compared to an isolated tumor cell in breast cancer (12).
Very rare cells, smaller than 8 µm, could be missed by filtration techniques. For example, in breast cancer, the size of CTC detected with CellSearch® system is comprised between 4 to 18 µm and obviously above 8-30 µm with filtration devices (13).
ISET® detects one tumor cell in 1 mL of peripheral blood (7,14). Several studies have compared the sensitivity of ISET® and CellSearch®. Farace et al. insisted on the superiority of ISET® compared to Cellsearch® in a series of 60 metastatic breast, prostate and lung cancer patients with 57 vs. 42 positive cases using ISET® and Cellsearch® respectively (15). A higher sensitivity of ISET® was demonstrated in hepatocellular carcinoma and in pancreatic cancer by Khoja et al. (16,17), in non-small cell lung cancer by Krebs et al. (13) and Hofman et al. (18). However, in these studies, CTCs were only detected in some patients by Cellsearch® and not by ISET®, probably corresponding to small CTCs.
Filtration devices allow selection of CTC on morphologic malignant criteria. Unlike this device, Cellsearch® defines CTC on immunological profile (EpCAM + cytokeratin + and CD45−) so benign epithelial cells, corresponding to these criteria, sometimes present in the blood stream could be misdiagnosed as CTCs. Therefore some false positive cases have been reported using this immunomagnetic technique (19).
False positivity also exists with filtration device due to the lack of specificity of this enrichment technique. Normal epidermal cells, which are collected by intra-dermic needle, can be present on the filter and misinterpreted as CTCs (they can be recognized with their large size and picnotic nuclei). Endothelial cells or rare hematologic cells such as megacaryocytes may also be present on the filter but are identified by pathologists according to their round and pale nucleus.
In some patients with no malignancy, circulating cells arising from parathyroid or thyroid adenoma have been described and can be collected on the filter; in this rare situation, these circulating cells disclose anisonucleosis, irregularity of the nuclear border, large nuclei and three-dimensional sheets which are very difficult to differentiate from true CTC (20). Naevic benign cells may also be present on the filter: De Giorgi et al. using ISET® filtration device reported the case of a patient with a congenital melanocytic benign nevus presenting binucleated and multinucleated circulating cells with malign criteria (21). In our experience (personal data) we detected some clusters of atypical cells using Screencell® device in patients with pancreatitis. Same detection of non-malignant circulating cells has been reported in benign pancreatic tumor (22).
Applications
Immunophenotyping
Immunocytochemical and immunofluorescent techniques can be performed on the filter in order to substantiate CTC nature or to characterize it. Many studies have used immunophenotyping to confirm the epithelial (23-25), melanocytic (26), hepatic (17), adrenocortical (27) or mesenchymal (28) nature of the suspected circulating cells. In our experience, CDX2 staining can be used to indicate CTC colorectal origin (Figure 2) (29). Most teams studied the epithelial-mesenchymal transition in the CTC using double immunostaining with a mesenchymal (vimentin, neural cadherin) and epithelial marker (16,18,30-32). Using this procedure, Hou et al. have shown that microemboli have a mesenchymal phenotype and have fewer images of apoptosis than isolated cells (31). Paul Hofman’s group performed BRAFV600E and ALK immunostaining in melanoma and lung adenocarcinoma respectively allowing identification of these theragnostic somatic mutations in CTC (33,34). Abdallah et al. performed thymidylate synthase immunostaining on CTC in patients with metastatic colorectal cancer; this enzyme is associated with 5-fluorouracile resistance (35). Numerous studies have confirmed the variability of immunophenotype among CTC confirming their heterogeneity (16,26,31,32). Using Ki67, a marker of all active phases of the cell cycle, it has been demonstrated that microemboli are non-proliferative unlike isolated cells (13). Detection of 5-aminolevulinic acid in CTC indicate presence of living CTC which are potentially responsible for metastasis (36).
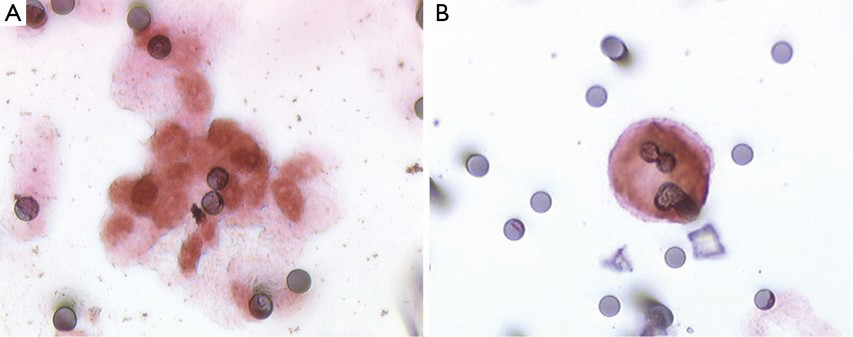
Culture
Specific devices are used for culture because of the necessity of collecting viable cells. Screencell® has developed filters with a lower pore size (6.5 µm) combined with an alternative dilution buffer for procedures not requiring fixation. The filter is then filed directly in a culture plate with classical culture medium. Cells were grown on the bottom of the well and on the filter. Recently, Metacell® has developed a size-based separation device driven by capillary-action (8). The filter is a polycarbonate membrane with 8 µm pores. Immediately after filtration, CTC are observed by light and fluorescent microscopy using unspecific fluorescent nuclear staining. Then, the filter maintained in a plastic ring is transferred to a culture plate.
Bobek et al. applied this technique in prostate (23), esophageal (24), mesothelioma (8), urothelial (37,38) and pancreas cancers (25) before performing immunostaining. Culture allows selection of viable CTCs and thus more tumor material in order to multiply downstream applications. However, culture could promote CTC dedifferentiation by selection of more viable clones. Screencell® also proposes a device for this application, but, to our knowledge, no results have been published yet.
Fluorescence in situ hybridization (FISH)
FISH can be performed on the filter with an adapted method. This method was applied in lung adenocarcinoma by the P. Hofman team in association with immunofluorescence in order to explore ALK rearrangement in CTC (34). Paillier et al. demonstrated that ALK-rearranged CTC harbored a mesenchymal phenotype and could represent a sub-cellular clone arising from a heterogeneous tumor (39). Awe et al. performed FISH on CTC with a telomeric probe: using a three-dimensional image acquisition system allowing the study of 3D telomeric organization reflecting chromosomic instability, they were able to assess degree of malignancy. Thus subpopulations of CTCs can be classified according to their 3D telomeric properties (40). Similarly, we performed FISH analysis in pancreatic cell line in our institution, in order to demonstrate polysomy (Figure 3). Indeed, in such settings, FISH can confirm the tumoral nature of CTCs in patients with pancreatic tumor.
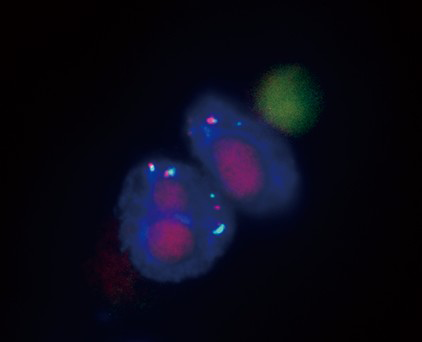
DNA analysis
Screencell®BM specific device has been commercialized to perform molecular biology. In this device, the filtration buffer is very similar to that of the culture device. The filter is a capsule adaptable on a collection tube. After filtration, lysis buffer is directly inser in the capsule-filter and products of lysis are then collecting in the tube by centrifugation. Then, classical steps of DNA or RNA extraction are performed.
However, if the number of the collected CTC is low, microdissection could be necessary to improve sensibility. In liver cancer, Vona et al. isolated CTCs from filter using laser microdissection capture: β-catenin gene mutations were analyzed by nested PCR followed by Sanger sequencing (41). Mutations were detected in 3 of 10 patients and only in a unique microdissected CTC for each patient emphasizing either technical bias or tumoral heterogeneity. Pinzani et al. measured HER-2 amplification by real-time PCR on breast cancer laser microdissected CTCs (14): 4 of the 7 patients presented HER-2 amplification in CTCs and in their matched primary tumors. Xu et al. employed a microfluidic platform using a slot pore microfilter to detect telomerase activity by real-time PCR-based telomeric repeat amplification protocol from filtered CTCs collected from metastatic prostate cancer patients (42).
But since demonstration of the feasibility of molecular biology techniques on CTCs isolated by filtration methods in 2000 (7), surprisingly, only few studies have shown results in patients. These difficulties are confirmed in our experience: in patients who presented KRAS mutated colon or pancreatic cancer, we failed to find exon 2 KRAS mutations in CTCs using SNaPshot® and QuantStudio® 3D Digital PCR System by Life Technologies® with or without microdissection. In these cases, we were only able to detect wild type KRAS sequences. One explanation for this lack of results could be the difficulties in performing these molecular techniques: they require a great number of steps on a very small number of CTCs. In the near future, this bias could be reduced using microfluidic devices. An alternative solution could be studying free circulating DNA: it appears that tumor circulating DNA allows easier and more reliable genomic analysis using new sensitive techniques such as digital PCR or next generation sequencing (43).
RNA analysis
Chen et al. using Screencell® filters studied epithelial-mesenchymal transition-related genes in CTCs arising in patient with prostate cancer (44): after complex micromanipulations in single isolated cell, they showed that genes promoting mesenchymal transition like IGF1, IGF2, EGFR, FOXP3, and TGFB3, were commonly expressed in CTCs, whereas epithelial genes were not.
Clinical interest
CTC as a prognostic biomarker
In two studies using filtration devices in adrenocortical and pancreatic cancers, CTC prognosis value was not reached; the low number of patients could explain this lack of conclusive prognostic value (16,45). However, Hofman et al. in their study on 204 patients with lung cancer, showed that the presence of CTCs detected by ISET® was an independent prognostic factor for shorter disease free survival (HR 1.372; 95% CI, 1.123-3.286%; P=0.006) (18). This team demonstrated that the presence of more than 50 CTCs was associated with shorter overall- and disease-free-survival (P=0.002; P=0.001) regardless of disease staging (46). In 31 patients with uveal melanoma, Mazzini et al. found a significant difference between overall- and disease-free-survival in stratifying groups of patients with more than 10 CTCs and with or without microemboli in univariate analysis (P=0.012; P=0.017) (47). In a series of 44 patients with hepatocellular carcinoma, Vona et al. demonstrated that presence (P=0.01) and number of CTCs and microemboli (P=0.02) correlated significantly with shorter survival (41). In a cohort of 131 patients with breast cancer, Wong et al. showed that in early breast cancer, there was an association between CTC level (CTC > or =4) and time to progression without statistical significance (P=0.05); in metastatic disease, median CTC ≥13 independently predicted time to progression (P=0.02) but no relationship between CTC level and overall survival was found in this subgroup (48).
Taken together, these studies emphasize that CTC isolation by filtration could be a good prognostic marker for several cancer localizations, but more studies with a greater number of cases are mandatory to consolidate this promising prognostic maker.
CTC in clinical follow up
Chinen et al. compared ISET® and a cytokeratin (CK)-dependent immunomagnetic separation method (Miltenyi®) in the follow-up of a patient with an undifferentiated lung cancer (32). Increase in CTC number correlated with worsening clinical status of the patient, while immunomagnetic technique showed a decrease in detected cells (maybe due to EMT phenomena). The authors suggested that filtration technique was superior to immunomagnetic separation in this clinical setting.
CTC as a predictive biomarker
Chen et al. in their study of CTC gene expression, showed that a subset of EMT-related gene was more expressed in castration-resistant prostatic cancers than in castration-sensitive cancers (44). So, a molecular signature could be interesting to predict castration sensitivity and thus optimize choice of therapeutic strategies. For theragnostic purposes, analysis of ALK translocation in lung cancer (34,39), BRAF mutation in melanoma (33) and HER-2 amplification in breast cancer (14) is feasible in CTCs. Thymidylate synthase CTC immunostaining is associated with metastatic colorectal cancer progression after 5-Fluorouracil treatment and therefore could predict resistance to this treatment (35).
Predictive makers can be detected using immunocytochemistry, therefore it is likely that these procedures are transposable to CTCs in a patient care setting. These approaches are promising because they could provide a more accessible source of tumor tissue compared to primitive or metastasis tumor tissues, allowing real-time monitoring of patient mutation status. Also CTC could be more representative of cancer heterogeneity than a fragment of tumor. But more studies with a greater number of cases are necessary to evaluate sensitivity, efficiency and real clinical benefit of these techniques.
CTC in diagnosis
CTC isolated by filtration could be of interest for the diagnosis of tumors which are difficult to sample. Our group has evaluated the diagnosis benefit of CTCs in pancreatic tumor (49). Sensitivity and specificity were 55.5% (95% CI, 40.1-70.9%) and 100% (95% CI, 75-100%), respectively. With a diagnosis accuracy of 70%, CTCs provide a noninvasive alternative to fine needle aspiration in pancreatic tumor. Hofman et al. studied CTC in 250 patients undergoing surgery for non-small cell lung carcinoma (50): 102 patients presented CTCs with malignant criteria before surgery with a low inter-observer variability. In another study on 76 broncho-pulmonary cancer patients, Freidin et al. found a 71.9.0% (95% CI, 60.5-83.0%) sensitivity and a 52.9% (95% CI, 31.1-77.0%) specificity of CTCs (51). A cohort of 77 patients with malignant and benign lung diseases was studied by Fiorelli et al. who showed that for a malignant circulating cell count greater than 25, sensitivity and specificity were 89% and 100%, respectively (52). In 72% of CTC positive patients, immunohistochemical analysis provides a specific histologic diagnosis of the corresponding primary tumor. Pinzani et al. provided the evidence that CTCs may represent a marker to support differential diagnosis between adrenocortical carcinoma and adrenocortical adenoma (27,45).
CTC detection could show an interest in cancer screening: indeed, there can be several months to years between initiation and detection of a tumor. CTCs can be present at the beginning of tumor development (22,53-55). Ilie et al. searched for CTCs in patients with chronic obstructive pulmonary disease which is a predisposing factor for lung cancer (56): five patients presented CTCs without any clinical or radiological proof of tumor and were annually followed by low-dose spiral computed tomography. For all these patients, lung nodules were detected 1 to 4 years after CTC detection, corresponding to early-stage lung cancer at histology. These sentinel CTCs could predict the occurrence of cancer in high-risk populations and could be used in tumor screening programs.
Perspectives: microfluidic devices
Size-selective microfluidic systems enable more precise and more efficient CTC enrichment than traditional filtration devices (57). Indeed, these nanotechnology structures, handling microliter amounts of liquid circulating in channels less than a tenth of a micrometer, allow more efficient CTC filtration (58-60). Furthermore microfluidic devices can facilitate downstream exploitation of CTCs (42,58,61). However, these new promising systems must be tested on large series of cases in order to validate their performance.
Conclusions
Filtration methods are sensitive and easy techniques which allow cytomorphological and immunocytochemical analysis of CTCs. However, due to the absence of multicentric studies, CTC clinical applications are not robust. Biological exploitations seem easy but are still difficult to develop requiring adaptation of known techniques for these very specific cells. Alternative free circulating DNA seems to be more accessible for molecular biology characterization. Developments of novel microfluidic techniques are promising and will, perhaps, allow greater clinical perspective.
Acknowledgements
The authors are grateful to Nikki Sabourin-Gibbs, Rouen University Hospital, for her help in editing the manuscript.
Footnote
Conflicts of Interest: The authors have no conflicts of interest to declare.
References
- Bockhorn M, Jain RK, Munn LL. Active versus passive mechanisms in metastasis: do cancer cells crawl into vessels, or are they pushed? Lancet Oncol 2007;8:444-8. [PubMed]
- Alix-Panabières C, Schwarzenbach H, Pantel K. Circulating tumor cells and circulating tumor DNA. Annu Rev Med 2012;63:199-215. [PubMed]
- Plaks V, Koopman CD, Werb Z. Cancer. Circulating tumor cells. Science 2013;341:1186-8. [PubMed]
- Seal SH. A sieve for the isolation of cancer cells and other large cells from the blood. Cancer 1964;17:637-42. [PubMed]
- Zabaglo L, Ormerod MG, Parton M, et al. Cell filtration-laser scanning cytometry for the characterisation of circulating breast cancer cells. Cytometry A 2003;55:102-8. [PubMed]
- Desitter I, Guerrouahen BS, Benali-Furet N, et al. A new device for rapid isolation by size and characterization of rare circulating tumor cells. Anticancer Res 2011;31:427-41. [PubMed]
- Vona G, Sabile A, Louha M, et al. Isolation by size of epithelial tumor cells: a new method for the immunomorphological and molecular characterization of circulatingtumor cells. Am J Pathol 2000;156:57-63. [PubMed]
- Bobek V, Kacprzak G, Rzechonek A, et al. Detection and cultivation of circulating tumor cells in malignant pleural mesothelioma. Anticancer Res 2014;34:2565-9. [PubMed]
- Chaffer CL, Weinberg RA. A perspective on cancer cell metastasis. Science 2011;331:1559-64. [PubMed]
- Mani SA, Guo W, Liao MJ, et al. The epithelial-mesenchymal transition generates cells with properties of stem cells. Cell 2008;133:704-15. [PubMed]
- Alix-Panabières C, Pantel K. Circulating tumor cells: liquid biopsy of cancer. Clin Chem 2013;59:110-8. [PubMed]
- Aceto N, Bardia A, Miyamoto DT, et al. Circulating tumor cell clusters are oligoclonal precursors of breast cancer metastasis. Cell 2014;158:1110-22. [PubMed]
- Krebs MG, Hou JM, Sloane R, et al. Analysis of circulating tumor cells in patients with non-small cell lung cancer using epithelial marker-dependent and -independent approaches. J Thorac Oncol 2012;7:306-15. [PubMed]
- Pinzani P, Salvadori B, Simi L, et al. Isolation by size of epithelial tumor cells in peripheral blood of patients with breast cancer: correlation with real-time reverse transcriptase-polymerase chain reaction results and feasibility of molecular analysis by laser microdissection. Hum Pathol 2006;37:711-8. [PubMed]
- Farace F, Massard C, Vimond N, et al. A direct comparison of CellSearch and ISET for circulating tumour-cell detection in patients with metastatic carcinomas. Br J Cancer 2011;105:847-53. [PubMed]
- Khoja L, Backen A, Sloane R, et al. A pilot study to explore circulating tumour cells in pancreatic cancer as a novel biomarker. Br J Cancer 2012;106:508-16. [PubMed]
- Morris KL, Tugwood JD, Khoja L, et al. Circulating biomarkers in hepatocellular carcinoma. Cancer Chemother Pharmacol 2014;74:323-32. [PubMed]
- Hofman V, Ilie MI, Long E, et al. Detection of circulating tumor cells as a prognostic factor in patients undergoing radical surgery for non-small-cell lung carcinoma: comparison of the efficacy of the CellSearch Assay™ and the isolation by size of epithelial tumor cell method. Int J Cancer 2011;129:1651-60. [PubMed]
- Pantel K, Denève E, Nocca D, et al. Circulating epithelial cells in patients with benign colon diseases. Clin Chem 2012;58:936-40. [PubMed]
- Hofman VJ, Ilie MI, Bonnetaud C, et al. Cytopathologic detection of circulating tumor cells using the isolation by size of epithelial tumor cell method: promises and pitfalls. Am J Clin Pathol 2011;135:146-56. [PubMed]
- De Giorgi V, Pinzani P, Salvianti F, et al. Circulating benign nevus cells detected by ISET technique: warning for melanoma molecular diagnosis. Arch Dermatol 2010;146:1120-4. [PubMed]
- Rhim AD, Thege FI, Santana SM, et al. Detection of circulating pancreas epithelial cells in patients with pancreatic cystic lesions. Gastroenterology 2014;146:647-51. [PubMed]
- Kolostova K, Broul M, Schraml J, et al. Circulating tumor cells in localized prostate cancer: isolation, cultivation in vitro and relationship to T-stage and Gleason score. Anticancer Res 2014;34:3641-6. [PubMed]
- Bobek V, Matkowski R, Gürlich R, et al. Cultivation of circulating tumor cells in esophageal cancer. Folia Histochem Cytobiol 2014;52:171-7. [PubMed]
- Bobek V, Gurlich R, Eliasova P, et al. Circulating tumor cells in pancreatic cancer patients: enrichment and cultivation. World J Gastroenterol 2014;20:17163-70. [PubMed]
- Khoja L, Shenjere P, Hodgson C, et al. Prevalence and heterogeneity of circulating tumour cells in metastatic cutaneous melanoma. Melanoma Res 2014;24:40-6. [PubMed]
- Scatena C, Pinzani P, Salvianti F, et al. Endocrine Pathology: SY08-1 detection of circulating tumor cells in adrenocortical neoplasms. Pathology (Phila) 2014;46 Suppl 2:S13-4.
- Chinen LT, Mello CA, Abdallah EA, et al. Isolation, detection, and immunomorphological characterization of circulating tumor cells (CTCs) from patients with different types of sarcoma using isolation by size of tumor cells: a window on sarcoma-cell invasion. Onco Targets Ther 2014;7:1609-17. [PubMed]
- Coget J, Borrini F, Susman S, et al. Colorectal carcinomas in 2013: the search for powerful prognostic markers is still on the go! Cancer Biomark 2014;14:145-50. [PubMed]
- Lecharpentier A, Vielh P, Perez-Moreno P, et al. Detection of circulating tumour cells with a hybrid (epithelial/mesenchymal) phenotype in patients with metastatic non-small cell lung cancer. Br J Cancer 2011;105:1338-41. [PubMed]
- Hou JM, Krebs M, Ward T, et al. Circulating tumor cells as a window on metastasis biology in lung cancer. Am J Pathol 2011;178:989-96. [PubMed]
- Chinen LT, de Carvalho FM, Rocha BM, et al. Cytokeratin-based CTC counting unrelated to clinical follow up. J Thorac Dis 2013;5:593-9. [PubMed]
- Hofman V, Ilie M, Long-Mira E, et al. Usefulness of immunocytochemistry for the detection of the BRAF(V600E) mutation in circulating tumor cells from metastatic melanoma patients. J Invest Dermatol 2013;133:1378-81. [PubMed]
- Ilie M, Long E, Butori C, et al. ALK-gene rearrangement: a comparative analysis on circulating tumour cells and tumour tissue from patients with lung adenocarcinoma. Ann Oncol 2012;23:2907-13. [PubMed]
- Abdallah EA, Fanelli MF, Buim ME, et al. Thymidylate synthase expression in circulating tumor cells: a new tool to predict 5-fluorouracil resistance in metastatic colorectal cancer patients. Int J Cancer 2015;137:1397-405. [PubMed]
- Matsusaka S, Kozuka M, Takagi H, et al. A novel detection strategy for living circulating tumor cells using 5-aminolevulinic acid. Cancer Lett 2014;355:113-20. [PubMed]
- Kolostova K, Cegan M, Bobek V. Circulating tumour cells in patients with urothelial tumours: Enrichment and in vitro culture. Can Urol Assoc J 2014;8:E715-20. [PubMed]
- Cegan M, Kolostova K, Matkowski R, et al. In vitro culturing of viable circulating tumor cells of urinary bladder cancer. Int J Clin Exp Pathol 2014;7:7164-71. [PubMed]
- Pailler E, Adam J, Barthélémy A, et al. Detection of circulating tumor cells harboring a unique ALK rearrangement in ALK-positive non-small-cell lung cancer. J Clin Oncol 2013;31:2273-81. [PubMed]
- Adebayo Awe J, Xu MC, Wechsler J, et al. Three-Dimensional Telomeric Analysis of Isolated Circulating Tumor Cells (CTCs) Defines CTC Subpopulations. Transl Oncol 2013;6:51-65. [PubMed]
- Vona G, Estepa L, Béroud C, et al. Impact of cytomorphological detection of circulating tumor cells in patients with liver cancer. Hepatology 2004;39:792-7. [PubMed]
- Xu T, Lu B, Tai YC, et al. A cancer detection platform which measures telomerase activity from live circulating tumor cells captured on a microfilter. Cancer Res 2010;70:6420-6. [PubMed]
- Bettegowda C, Sausen M, Leary RJ, et al. Detection of circulating tumor DNA in early- and late-stage human malignancies. Sci Transl Med 2014;6:224ra24.
- Chen CL, Mahalingam D, Osmulski P, et al. Single-cell analysis of circulating tumor cells identifies cumulative expression patterns of EMT-related genes in metastatic prostate cancer. Prostate 2013;73:813-26. [PubMed]
- Pinzani P, Scatena C, Salvianti F, et al. Detection of circulating tumor cells in patients with adrenocortical carcinoma: a monocentric preliminary study. J Clin Endocrinol Metab 2013;98:3731-8. [PubMed]
- Hofman V, Bonnetaud C, Ilie MI, et al. Preoperative circulating tumor cell detection using the isolation by size of epithelial tumor cell method for patients with lung cancer is a new prognostic biomarker. Clin Cancer Res 2011;17:827-35. [PubMed]
- Mazzini C, Pinzani P, Salvianti F, et al. Circulating tumor cells detection and counting in uveal melanomas by a filtration-based method. Cancers (Basel) 2014;6:323-32. [PubMed]
- Wong NS, Kahn HJ, Zhang L, et al. Prognostic significance of circulating tumour cells enumerated after filtration enrichment in early and metastatic breast cancer patients. Breast Cancer Res Treat 2006;99:63-9. [PubMed]
- Iwanicki-Caron I, Basile P, Toure E, et al. Usefulness of circulating tumor cell detection in pancreatic adenocarcinoma diagnosis. Am J Gastroenterol 2013;108:152-5. [PubMed]
- Hofman V, Long E, Ilie M, et al. Morphological analysis of circulating tumour cells in patients undergoing surgery for non-small cell lung carcinoma using the isolation by size of epithelial tumour cell (ISET) method. Cytopathology 2012;23:30-8. [PubMed]
- Freidin MB, Tay A, Freydina DV, et al. An assessment of diagnostic performance of a filter-based antibody-independent peripheral blood circulating tumour cell capture paired with cytomorphologic criteria for the diagnosis of cancer. Lung Cancer 2014;85:182-5. [PubMed]
- Fiorelli A, Accardo M, Carelli E, et al. Circulating Tumor Cells in Diagnosing Lung Cancer: Clinical and Morphologic Analysis. Ann Thorac Surg 2015;99:1899-905. [PubMed]
- Chang YS, di Tomaso E, McDonald DM, et al. Mosaic blood vessels in tumors: frequency of cancer cells in contact with flowing blood. Proc Natl Acad Sci U S A 2000;97:14608-13. [PubMed]
- Paterlini-Brechot P, Benali NL. Circulating tumor cells (CTC) detection: clinical impact and future directions. Cancer Lett 2007;253:180-204. [PubMed]
- Rhim AD, Mirek ET, Aiello NM, et al. EMT and dissemination precede pancreatic tumor formation. Cell 2012;148:349-61. [PubMed]
- Ilie M, Hofman V, Long-Mira E, et al. "Sentinel" circulating tumor cells allow early diagnosis of lung cancer in patients with chronic obstructive pulmonary disease. PLoS One 2014;9:e111597. [PubMed]
- Sun W, Jia C, Huang T, et al. High-performance size-based microdevice for the detection of circulating tumor cells from peripheral blood in rectal cancer patients. PLoS One 2013;8:e75865. [PubMed]
- Lee A, Park J, Lim M, et al. All-in-one centrifugal microfluidic device for size-selective circulating tumor cell isolation with high purity. Anal Chem 2014;86:11349-56. [PubMed]
- Hosokawa M, Yoshikawa T, Negishi R, et al. Microcavity array system for size-based enrichment of circulating tumor cells from the blood of patients with small-cell lung cancer. Anal Chem 2013;85:5692-8. [PubMed]
- Sollier E, Go DE, Che J, et al. Size-selective collection of circulating tumor cells using Vortex technology. Lab Chip 2014;14:63-77. [PubMed]
- Riahi R, Gogoi P, Sepehri S, et al. A novel microchannel-based device to capture and analyze circulating tumor cells (CTCs) of breast cancer. Int J Oncol 2014;44:1870-8. [PubMed]