Heat shock protein inhibitor, quercetin, as a novel adjuvant agent to improve radiofrequency ablation-induced tumor destruction and its molecular mechanism
Introduction
Percutaneous local ablative therapy has emerged as a safe and effective treatment for small solid tumors (1-3). Among the various percutaneous local ablative therapies, radiofrequency ablation (RFA) has garnered the greatest interest because of its effectiveness and safety for small hepatocellular carcinomas (HCCs) ≤5.0 cm, with a 3-year survival rate of 62–68%, a low complication rate of 0–12%, and a low treatment mortality of 0–1% (4). However, a key limitation remains the inefficient treatment of larger tumors, which often leads to local tumor recurrence (5). It was recently reported that following RFA-mediated tumor ablation in HCC patients, an 8-fold increase in heat shock protein 70 (HSP70) and a 1.2-fold increase in HSP90 occur in the cytoplasm and membranes of tumor cells (6). Consistently, multiple animal studies have confirmed significant increase in HSP70 levels after RFA, and thereby limited the therapeutic effects (7,8).
A number of small molecular inhibitors of heat-induced HSP70 expression have been reported. The most effective one of these inhibitors is quercetin (3,3',4',5,7-pentahydroxyflavone), which is benzylidene lactam compound (9,10). Recently, quercetin was reported to induce apoptosis in X. laevis A6 cells through down-regulation of HSPs (11). Quercetin is not only as effective as a single agent but also enhances the cytotoxic effect of the chemotherapeutic agent, paclitaxel, in combinatorial therapy, which appears to regulate HSP70 expression through an unknown mechanism(s) (12).
Here, we reported that the combination of RFA with adjuvant quercetin-loaded liposomes increases tumor coagulation and survival by inhibiting HSP70 through a mechanism that appears to require heat shock factor 1 (HSF1). More importantly, the suppression of HSF1 improved the outcome of combination therapy. Interestingly, the combination of quercetin with RFA increased the treatment efficacy in our cancer model, and this effect appeared to require activating protein-1 (AP-1) signaling pathways. Our work presents the novel idea of combining quercetin treatment with RFA to improve the clinical outcome of cancer therapy.
Materials and methods
We used the HSP70 inhibitor, quercetin, loaded in liposomes to increase the local concentration in combination with RFA and investigated whether there were any improvements in the treatment outcomes in an animal model with breast tumor and the potential molecular mechanisms of effects in an animal model and human cancer cells.
Animal models
All animal studies were performed in accordance with the regulations of the Institutional Animal Care and Use Committee of Peking University Oncology School and approval was granted prior to the initiation of the study. Anesthesia was always induced via the intraperitoneal injection of pentobarbital sodium (45 mg/kg, Foshan, China). The animals were sacrificed in a CO2 chamber. The RFA experiments were performed using a well-characterized (13) and established R3230 mammary adenocarcinoma cell line that was kindly provided by the minimally invasive tumor therapy lab of Beth Israel Deaconess Medical Center, MA. Male Fisher rats (100±20 g; 7–8 weeks old, Vital River Laboratory Animal Technology Co., Ltd., China.) with R3230 tumors were used for the in vivo studies. A single tumor was implanted into each animal via the slowly injection of 0.3–0.4 mL of tumor cell suspension into the mammary fat pad of each animal using an 18-gauge needle. The animals were monitored every 2–3 d to measure tumor growth. Solid non-necrotic tumors 1.2–1.5 cm in diameter (as determined by ultrasonography at the time of treatment) were used for this study. The survival endpoint was a maximum tumor diameter of 30 mm or a survival of 90 d, whichever was reached first.
In total, 80 rats were used in this study. The rats were randomized into four treatment arms [control, quercetin alone, radiofrequency (RF) alone, and combination of RF and quercetin) for outcome analysis (n=10 each group) and for Kaplan-Meier analysis of the defined survival endpoint (n=10).
Radiofrequency ablation procedure
In the animal study, standard monopolar RFA was applied by using a 480-kHz RFA generator (Model CC-1-220; Valleylab, Tyco Healthcare, USA). Initially, the 0.7 cm tip of a 17-gauge electrically insulated electrode (ACT1507 electrode; Valleylab, Tyco Healthcare, USA) was placed in the center of the tumor. RF was applied for 5 min with the generator output titrated to maintain a designated tip temperature (70±2 °C).
Preparation and administration of adjuvant liposomal quercetin
Polyethylene glycol (PEG)-modified liposomal quercetin was prepared in a pharmaceutical preparation laboratory and described in previous studies (13). Liposomal quercetin was administered slowly via tail vein injection. The combination group received a 24 h pre-RFA along with quercetin at the maximum liposomal saturation dose (0.029 mg/mL).
HSF1-deficient tumor animal model
HSP expression was inhibited by targeting the transcription factor HSF1, which is a common upstream regulator of HSP family genes, using short hairpin RNA (shRNA) (Sigma, Germany) packaged into lentivirus to infect target R3230 cells. Control cells were infected with lentivirus carrying scrambled shRNA sequences. Western blot analyses were subsequently performed to determine the efficiency of the knockdown of HSF1 expression, and confirmation was performed with immunofluorescence (IF) staining. To study the in vivo effects, an HSF1-deficient rat model was developed, and two different tumor cell suspensions were injected into the mammary fat pad to further study and characterize the HSF1-deficient and control tumors (n=5, each group).
Tumor necrosis characterization
The rats were sacrificed 24 h after ablation. Half of each tumor was incubated in 2,3,5-triphenyltetrazolium chloride (TTC, 2%; Sigma, Germany) solution for 15–20 min at 37 °C. Previous studies have documented close correlations between gross pathological and histopathological findings regarding RF-induced coagulation necrosis (13). The area of necrosis was taken as the area unstained by TTC on the largest section. The tumor coagulated area was calculated as follows: area = [(longitude diameter+width diameter)/2]2×3.14. The remaining half of the sections were transferred into 10% formaldehyde solution for fixation followed by appropriate staining/imaging.
Immunohistochemistry (IHC) and IF analyses
The tissues were stained with hematoxylin and eosin (H&E) for gross pathological examination. IHC and IF staining were performed using antibodies against HSF1 (Cell Signaling Technology, Danvers, MA, USA), HSP70 (Stressgen, Chicago, IL, USA), and cleaved caspase-3 (Cell Signaling Technology, Danvers, MA, USA) with previously described techniques (13).
Cell culture
The in vitro experiments involved the culturing of human breast adenocarcinoma cell line (MCF-7, HTB-22, ATCC) and human hepatocellular carcinoma cell line (SK-HEP-1, HTB-52, ATCC) in Dulbecco’s Modified Eagle’s Medium (DMEM, ATCC) supplemented with 10% fetal bovine serum (FBS, Invitrogen). The cells were seeded on coverslips at a density of 1×106 cells/mL (for indirect IF) and incubated at 37 °C under a humidified atmosphere with 5% CO2.
Heat shock and drug treatment cell model
In the in vitro experiments, lyophilized quercetin (Sigma, Germany) dissolved in dimethyl sulfoxide (DMSO, Sigma, Germany) was used in different concentrations. Four variants of the experiments were performed. First, hyperthermia of the cells was induced by exposing to different temperatures (42, 45 or 50 °C) for 1 h, and HSP70 expression was then tested with Western blotting. Second, the cells were exposed to hyperthermia-inducing conditions (42 °C) for different duration (2, 6, or 12 h), and HSP70 expression was then tested with Western blotting. Third, the cell cultures were pre-incubated with quercetin in different concentrations (10, 30, or 50 µmol/L) for 1 h at 37 °C and then exposed to hyperthermia-inducing conditions (42 °C for 6 h). HSP70 expression was then tested with Western blotting. Fourth, the cultures were pre-incubated with quercetin for different duration (0, 1, 2, or 6 h) at 37 °C and then exposed to hyperthermia-inducing conditions (42 °C for 6 h). HSP70 expression was then tested with Western blotting. As controls, cells were incubated with 0.1% DMSO at 37 or 42 °C. The heat shock model and quercetin treatment parameters were based on our earlier experiments and observations by other authors (14,15). Based on these four experiments, we used the condition in which the cells were pre-incubated with quercetin at 50 µmol/L for 1 h and exposed to hyperthermia-inducing conditions (42 °C for 6 h) as the optimized heat shock and drug treatment model for the following introductory experiments.
Western blotting analysis
Using the previously described technique (13), protein contents of tumor samples were measured using densitometry analysis and normalized for equal loading using the β-actin protein content (Cell Signaling Technology, Danvers, MA, USA). The primary antibodies included Phospho-c-Jun (Ser63) II antibody (Cell Signaling Technology), c-Jun (Cell Signaling Technology), HSF1 (Cell Signaling Technology), HSP70 (Stressgen, Chicago, MI, USA), HSP90 (Stressgen, Chicago, USA), cleaved caspase-3 (Cell Signaling Technology), and caspase-3 (Cell Signaling Technology).
Reporter assays
The pGL2-AP-1-Luc luciferase reporter plasmids (generously given by Dr. Zhu and Rosenfeld) were generated by cloning AP-1 response elements cloned into the pGL2-Luc plasmid (16). MCF-7 or SK-HEP-1 cells (2×104 each well) were transfected with the pGL2-AP-1-Luc luciferase reporter plasmid using Lipofectamine 2000 (Invitrogen, Carlsbad, CA, USA).
Statistical analysis
Data are expressed as
Results
HSP70 inhibitor improved RF therapeutic outcome in vivo
Our data indicated that the combination of liposomal quercetin and RF (503±39 mm2) resulted in a significant increase in tumor destruction compared with liposomal quercetin (20±9 mm2) and RF therapy alone (225±36 mm2; P<0.01 for all comparisons; Figure 1A,B). Furthermore, the combination of liposomal quercetin and RF therapy significantly increased the endpoint survival in the rat tumor model largely due to decreases in the tumor growth rate (Figure 1C,D). The endpoint survivals were 12.3±2.4 d for the control group, 17.6±2.5 d for the RF alone group, 15.7±3.1 d for the liposomal quercetin alone group, and 26.5±3.4 d for the combined RF and quercetin group (P<0.05 for all comparisons).
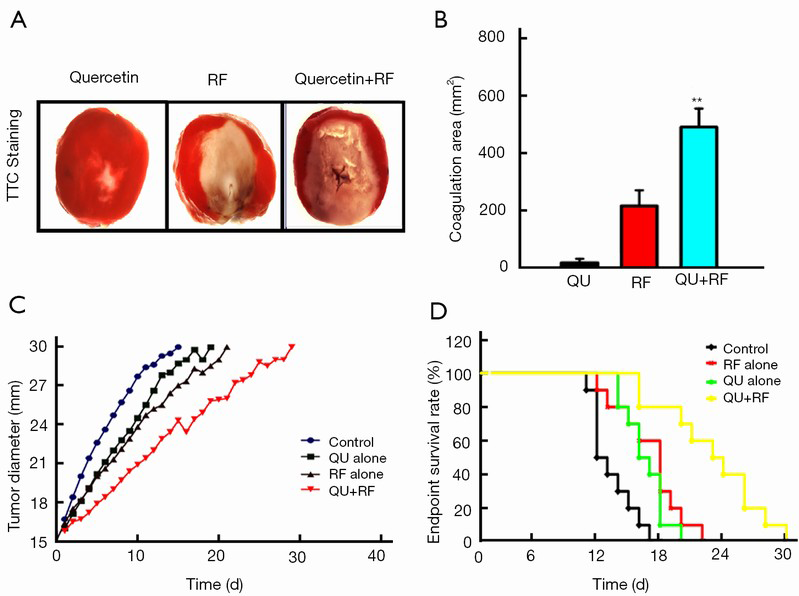
Quercetin suppressed HSP70 accumulation in tumors after combination therapy and promoted cell apoptosis via HSF1 pathway
Next, we investigated whether HSP70 was the key factor limiting the RF therapy range in the animal model. To address this issue, we monitored caspase-3 and HSP70 expression in the post-treatment tumor samples and found that HSP70 levels increased significantly in the coagulation edge in the RF alone group at 24 h after RF. More interestingly, cleaved caspase-3 was located closely inside the HSP70 rim, which suggested that HSP70 blocked cancer cell death upon RF therapy. Interestingly, quercetin significantly decreased HSP70 expression in the combination group compared with RF alone group. Furthermore, caspase-3 expression significantly increased in the combination group, compared with the RF alone group (Figure 2A). These data suggested that quercetin increased the efficacy of RF by increasing apoptosis. It is well known that HSF1 is a transcription factor with critical roles in the regulation of HSP expression. Therefore, next, we investigated HSF1 expression in different treatment groups and observed significantly reduced HSF1 expression in the combination group, compared with the RF alone group (Figure 2B).
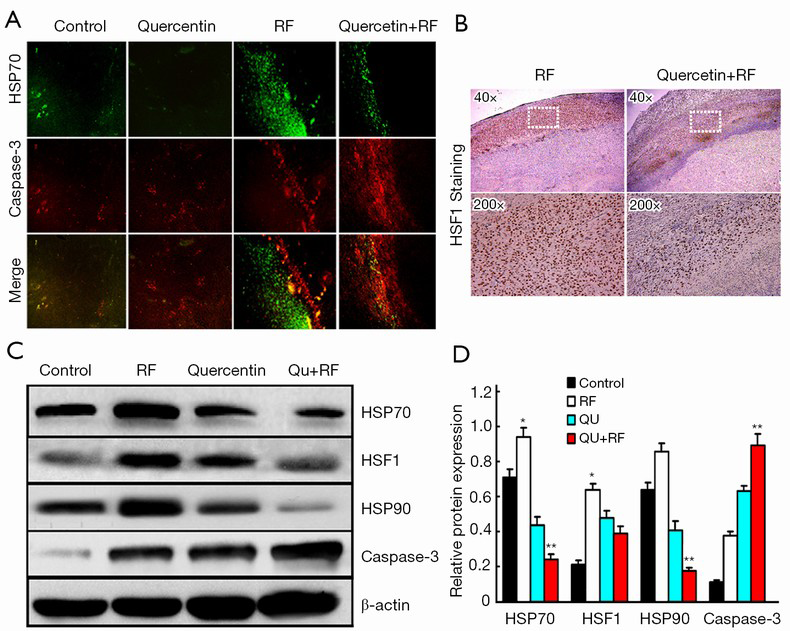
To confirm the changes in HSP70, HSP90, caspase-3 and HSF1 expressions in the pathological state, we examined the levels of these proteins in tumors of the various treatment groups. HSP70 and HSP90 were significantly decreased in the combination group compared with the RF alone group. Consistently, the HSF1 level was significantly decreased in the combination group, whereas caspase-3 expression was strikingly increased (Figure 2C,D).
HSF1 activation was critical for improved RF efficiency
To investigate whether HSF1 was critical to the improved therapeutic efficacy of combination therapy, we performed the RF treatment in an HSF1-deficient animal model. First, we confirmed the significantly reduced/absent levels of HSF1 in the tumor samples obtained from the HSF1-deficient rat model (data not shown). Furthermore, our findings revealed that the RF coagulation area was significantly increased in the HSF1-deficient tumor model (498±42 vs. 268±31 mm2, P<0.001), compared with the control at 24 h after RF (Figure 3A,B). Next, IF and IHC staining analyses confirmed the reduced expression of HSP70 in the HSF1-deficient tumor model, however, the expression of caspase-3 increased (Figure 3C).
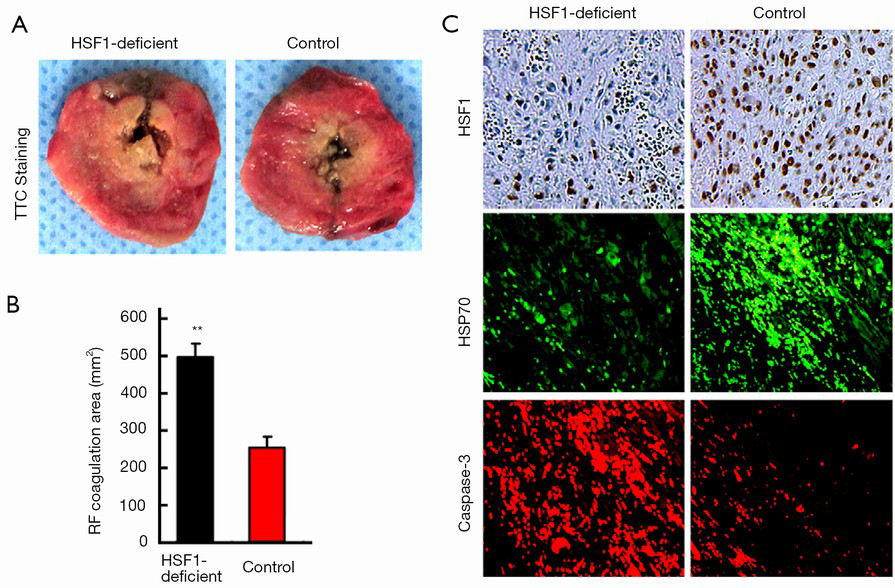
Quercetin increased apoptosis in human liver and breast cancer cells after heat shock
We used a heat shock cell model and studied the changes in HSP70 and cleaved caspase-3 expression with and without quercetin incubation in the breast and liver cancer cell lines. Our results revealed a significant reduction in HSP70 and an increase in caspase-3 levels in MCF-7 cells exposed to the combination of heat shock and quercetin compared with the heat shock alone. These findings were consistent with those in SK-HEP-1 liver cancer cells (Figure 4A,B). Consistently, HSP70 expression exhibited both time and dose dependence in the heat shock cell model as confirmed by Western blotting (Figure 4C-E).
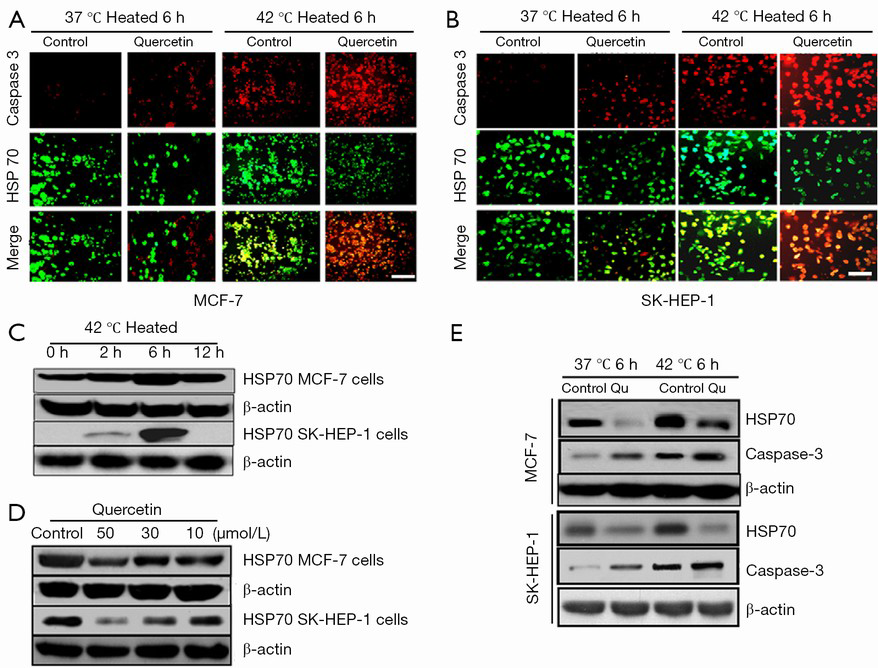
Inhibition of HSF1 by quercetin involved AP-1 signaling pathways
To assess the effect of quercetin, if any, on the signaling pathways that are known to regulate HSF1 activity, we analyzed the expressions of the phospho-c-Jun (p-c-Jun) and c-Jun (i.e., AP-1 components) in the tumor samples from the rat tumor model and the human cancer cells (MCF-7 and SK-HEP-1) challenged with quercetin in vitro in the heat shock cell model. We observed significantly lower levels of p-c-Jun in tumors receiving the combination therapy compared with RF or quercetin alone (Figure 5A). Consistently, in the human cancer cells (MCF-7), quercetin exposure also resulted in a significant reduction in p-c-Jun compared with vehicle alone in vitro (Figure 5B). To directly evaluate whether quercetin regulated the activity of AP-1, we used a firefly luciferase reporter gene (under the control of AP-1 response elements) assay to measure any direct effects of quercetin treatment on the transcriptional output of MCF-7 cancer cells. As illustrated in Figure 5C, D, the quercetin treatment resulted in significantly reduced HSF1 and AP-1 transcriptional activities compared with the vehicle treatment, suggesting that quercetin exposure regulated the transcriptional output of tumor cells through down-regulation of AP-1 activity.
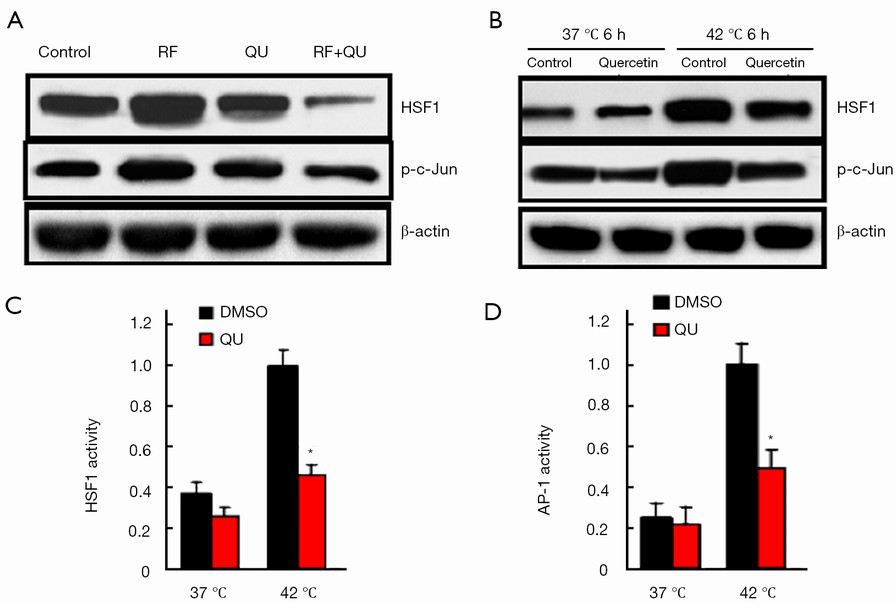
Discussion
In the present study, we reported that combining RFA with adjuvant quercetin-loaded liposomes increased tumor cell death by limiting the actions of transcription factor HSF1 and subsequently inhibiting HSP70 and thereby resulting in improved clinical outcomes. Consistently, blocking HSF1 in vitro (via siRNA mediated knockdown) improved the therapeutic outcomes when used in combination with heat. Intriguingly, the actions of quercetin that improved the clinical outcomes in the combination therapy with RF appeared to require the AP-1 signaling pathways. Recently, the AP-1 signal pathways were found to be closely related to cancer invasion and metastasis and have thus drawn increasing attention in the oncology research field. Lin et al. reported that quercitin could significantly suppressed c-Jun expression accompanied by a reduction in AP-1 transcriptional activity and therefore inhibited MMP-9 gene expression (17). These results provided insight into the novel signaling pathways, through which, drugs such as quercitin, may act to improve the clinical outcomes of cancer patients when used in combination with existing therapies, including RFA, and provided new avenues for improving existing clinical strategies.
Combination of quercetin and radiofrequency ablation in tumor therapy
Recently, the use of RFA for neoplasms arising in the liver, bone, lung, breast, and kidney has been the focus of much studies (18,19). However, additional work is required to overcome the difficulties associated with the achievements of complete ablative margins for larger tumors. One of the main factors associated with this limitation during the course of cancer treatment is the induction of the cellular stress response via the up-regulation of HSP family members, which limits the clinical outcomes of RF treatment, particularly in liver cancer. Therefore, strategies that can increase the tumor destruction extent of RF are desired. One such successful strategy has been the targeting of residual viable cells with adjuvant chemotherapy or radiation to improve the completeness of RFA (20,21).
HSPs and tumor therapy
HSPs are overexpressed in a range of human carcinomas, including both solid tumors and hematological malignancies (22,23). A number of animal studies have confirmed that HSP70 is indeed significantly increased after RFA (24), which limits tumor tissue damages. Furthermore, HSP70 can also protect cells against apoptosis via several mechanisms, such as blocking cytochrome C release from mitochondria, and inhibiting procaspase-9 activation, apoptosome formation and c-Jun N-terminal kinase (JNK) phosphorylation (25,26). Consistently, in our study, we demonstrated how HSP70 was greatly induced in a R3230 tumor model upon RFA. Moreover, the HSP70 concentration in the RFA area ring was striking, and this expression overlapped well with the reduction in apoptosis or tumor cell death. These data suggest that HSPs have the potential to be used as key therapeutic targets in improving the clinical outcomes of tumors treated with RFA.
Quercetin-induced cancer cell death is dependent on HSPs and their downstream effectors
Quercetin has recently garnered immense interest as a potent inducer of cancer cell death in vivo and in vitro. Recently, in vitro studies have also revealed that the growth of cultured skin and prostate cancer cells is suppressed upon treatment with a combination of quercetin and radiation (15). For example, a recent study demonstrated that quercetin is associated with a 23% reduction in the risk of pancreatic cancer, which is a rare but frequently fatal disease. Due to these benefits that have observed in clinical studies in humans, quercetin appears to be a promising pharmacological agent for therapeutic interventions. Although the survival benefits of quercetin have been well documented in our work and previous reports, the mechanism(s) of its action has remained largely unknown.
To this end, we investigated the mechanisms of quercetin action in the present study and attempted to identify novel signaling pathways and drug targets that can be used in clinic to improve the survival of patients with breast or liver cancer. Using a heat shock-induced rat model, we reported that quercetin treatment was accompanied by a reduced expression of HSP70 that led to the reduced function of its downstream effector HSF1, which in turn led to the increase in cancer cell death. Further investigations revealed that the critical role of AP-1 pathway in the regulation of the quercetin-induced reduction in HSP70 led to the down-regulation of HSF1 and thereby caused cancer cell death. Interestingly, in the RFA model, quercetin mediated the deceleration of tumor development via HSP70 suppression, and this effect appeared to be partially due to the subsequent inactivation of its downstream effector protein AP-1. We believed that this pathway is an interesting target for the design of novel cancer therapeutics used in combination with existing therapies.
Conclusions
Finally, our findings revealed a novel signaling pathway that involves AP-1-HSF1/HSP70 regulation and is influenced by quercetin treatment to improve the therapeutic efficacy of solid cancers. Hence, these results present a unique opportunity for the targeting of this pathway in the design of better therapeutic strategies for using in combination with RFA.
Acknowledgements
This work was supported by the National Natural Science Foundation of China (Commission No. 81471768). W Yang was supported by Beijing Municipal Health System Special Funds of High-Level Medical Personnel Construction (No. 2013-3-086).
Footnote
Conflicts of Interest: The authors have no conflicts of interest to declare.
References
- Zheng RN, You ZJ, Lin SH, et al. Efficacy of percutaneous radiofrequency ablation for the treatment of hepatocellular carcinoma. Genet Mol Res 2015;14:17982-94. [PubMed]
- Zhang F, Chang X, Liu T, et al. Prognostic Factors for Long-Term Survival in Patients with Renal-Cell Carcinoma After Radiofrequency Ablation. J Endourol 2016;30:37-42. [PubMed]
- Chu KF, Dupuy DE. Thermal ablation of tumours: biological mechanisms and advances in therapy. Nat Rev Cancer 2014;14:199-208. [PubMed]
- Liang HH, Chen MS, Peng ZW, et al. Percutaneous radiofrequency ablation versus repeat hepatectomy for recurrent hepatocellular carcinoma: a retrospective study. Ann Surg Oncol 2008;15:3484-93. [PubMed]
- Livraghi T, Goldberg SN, Lazzaroni S, et al. Hepatocellular carcinoma: radio-frequency ablation of medium and large lesions. Radiology 2000;214:761-8. [PubMed]
- Schueller G, Kettenbach J, Sedivy R, et al. Expression of heat shock proteins in human hepatocellular carcinoma after radiofrequency ablation in an animal model. Oncol Rep 2004;12:495-9. [PubMed]
- Bhardwaj N, Dormer J, Ahmad F, et al. Heat shock protein 70 expression following hepatic radiofrequency ablation is affected by adjacent vasculature. J Surg Res 2012;173:249-57. [PubMed]
- Solazzo SA, Ahmed M, Schor-Bardach R, et al. Liposomal doxorubicin increases radiofrequency ablation-induced tumor destruction by increasing cellular oxidative and nitrative stress and accelerating apoptotic pathways. Radiology 2010;255:62-74. [PubMed]
- Powers MV, Workman P. Targeting of multiple signalling pathways by heat shock protein 90 molecular chaperone inhibitors. Endocr Relat Cancer 2006;13 Suppl 1:S125-35. [PubMed]
- Choi JA, Kim JY, Lee JY, et al. Induction of cell cycle arrest and apoptosis in human breast cancer cells by quercetin. Int J Oncol 2001;19:837-44. [PubMed]
- Manwell LA, Heikkila JJ. Examination of KNK437- and quercetin-mediated inhibition of heat shock-induced heat shock protein gene expression in Xenopus laevis cultured cells. Comp Biochem Physiol A Mol Integr Physiol 2007;148:521-30. [PubMed]
- Chang YF, Hsu YC, Hung HF, et al. Quercetin induces oxidative stress and potentiates the apoptotic action of 2-methoxyestradiol in human hepatoma cells. Nutr Cancer 2009;61:735-45. [PubMed]
- Yang W, Ahmed M, Tasawwar B, et al. Combination radiofrequency (RF) ablation and IV liposomal heat shock protein suppression: reduced tumor growth and increased animal endpoint survival in a small animal tumor model. J Control Release 2012;160:239-44. [PubMed]
- Chang YF, Chi CW, Wang JJ. Reactive oxygen species production is involved in quercetin-induced apoptosis in human hepatoma cells. Nutr Cancer 2006;55:201-9. [PubMed]
- Paliwal S, Sundaram J, Mitragotri S. Induction of cancer-specific cytotoxicity towards human prostate and skin cells using quercetin and ultrasound. Br J Cancer 2005;92:499-502. [PubMed]
- Perissi V, Aggarwal A, Glass CK, et al. A corepressor/coactivator exchange complex required for transcriptional activation by nuclear receptors and other regulated transcription factors. Cell 2004;116:511-26. [PubMed]
- Lin CW, Hou WC, Shen SC, et al. Quercetin inhibition of tumor invasion via suppressing PKC delta/ERK/AP-1-dependent matrix metalloproteinase-9 activation in breast carcinoma cells. Carcinogenesis 2008;29:1807-15. [PubMed]
- Ahmed M, Brace CL, Lee FT Jr, et al. Principles of and advances in percutaneous ablation. Radiology 2011;258:351-69. [PubMed]
- Park S, Cadeddu JA. Outcomes of radiofrequency ablation for kidney cancer. Cancer Control 2007;14:205-10. [PubMed]
- Moussa M, Goldberg SN, Kumar G, et al. Radiofrequency ablation-induced upregulation of hypoxia-inducible factor-1α can be suppressed with adjuvant bortezomib or liposomal chemotherapy. J Vasc Interv Radiol 2014;25:1972-82. [PubMed]
- Horkan C, Dalal K, Coderre JA, et al. Reduced tumor growth with combined radiofrequency ablation and radiation therapy in a rat breast tumor model. Radiology 2005;235:81-8. [PubMed]
- Calderwood SK. Heat shock proteins in breast cancer progression--a suitable case for treatment? Int J Hyperthermia 2010;26:681-5. [PubMed]
- Soo ET, Yip GW, Lwin ZM, et al. Heat shock proteins as novel therapeutic targets in cancer. In Vivo 2008;22:311-5. [PubMed]
- Yang W, Ahmed M, Elian M, et al. Do liposomal apoptotic enhancers increase tumor coagulation and end-point survival in percutaneous radiofrequency ablation of tumors in a rat tumor model? Radiology 2010;257:685-96. [PubMed]
- Xanthoudakis S, Nicholson DW. Heat-shock proteins as death determinants. Nat Cell Biol 2000;2:E163-5. [PubMed]
- Gabai VL, Yaglom JA, Volloch V, et al. Hsp72-mediated suppression of c-Jun N-terminal kinase is implicated in development of tolerance to caspase-independent cell death. Mol Cell Biol 2000;20:6826-36. [PubMed]