BMPR2 and HIF1-α overexpression in resected osteosarcoma correlates with distant metastasis and patient survival
Introduction
Osteosarcoma is the most common primary malignant bone tumor. It mainly occurs in children and adolescents and is characterized by local aggressiveness and high rates of lung metastasis (1). It is reported that approximately 20% of osteosarcoma patients exhibit lung metastasis at diagnosis, and for the rest, 2 out of 5 patients were diagnosed with metastatic lesions at a later stage (2). With the refinement of multimodality treatments, including neoadjuvant chemotherapy, surgical techniques and radiotherapy, in the most recent two decades, the five-year survival of patients with localized osteosarcoma has improved (3). However, the five-year survival of patients with lung metastases is only 28% (4). The clinical outcomes of osteosarcoma patients with or without lung metastasis are significantly varied. Therefore, identifying metastasis-associated molecules is of great clinical significance because of the poor prognosis of osteosarcoma patients with lung metastasis.
Bone morphogenetic protein receptor 2 (BMPR2) belongs to the transforming growth factor-β (TGF-β) superfamily of proteins (5). As the primary binding protein of mature bone morphogenetic protein (BMP) ligands, BMPR2 contains three domains: a short extracellular binding one, a transmembrane one and an intracellular one with serine/threonine kinase activity (5). Classical BMP signaling begins with the combination of BMP proteins and the heterotetrameric complex (consisted of BMPR1 and BMPR2), which leads to the activation of Smad molecules. In addition, BMPR2 also participates in the Smad-independent pathway, which involves other different intracellular modulators, such as PI3K, p38 MAPK, and ERK (6). Many researches have demonstrated that BMPR2 is involved in the progression of tumors. However, the role of BMPR2 in different tumors remains controversial. BMPR2 serves as a tumor suppressor gene in human prostate cancer (7) and colon polyps (8). But in breast cancer (9) and chondrosarcoma cells (10), BMPR2 functions as an oncogene. While in human osteosarcoma cells, the protein expression and concrete mechanism of BMPR2 remain veiled.
Intratumoral hypoxia is a common characteristic of solid malignancies, including sarcomas (11-13). Hypoxia-inducible factor 1-α (HIF1-α), an indispensable transcription factor for the cellular adaptation to hypoxia, is related to tumor initiation and progression (14-16). Previous studies showed that the invasive capacity of osteosarcoma cells was enhanced under hypoxic conditions (17). However, a link between BMPR2 and HIF1-α has not been investigated. This study discusses the expression of BMPR2 and HIF1-α in human osteosarcoma samples and emphasizes the role of the two markers in the prognosis of osteosarcoma patients.
Materials and methods
Patients and samples
Ethical approval in the present study was obtained from the ethics committee of Peking University People’s Hospital (Approved number: 2015-60, December 2015). Written informed consent was also obtained from all patients or their guardians. We examined the medical records of osteosarcoma patients between January 2004 and May 2014 at the Musculoskeletal Tumor Center of Peking University People’s Hospital (Beijing, China). A total of 103 tissue specimens from osteosarcoma patients who underwent osteosarcoma resection were included in our research. None of the patients underwent pre-operative radiotherapy, and no tumor metastasis occurred at the time of diagnosis. The following paraffin-embedded specimens were obtained from the Department of Pathology in Peking University People’s Hospital. Two experienced pathologists independently carried out the diagnosis of all specimens and they came to an agreement after discussion and consensus.
Follow-up
To observe the status of patient survival and metastasis, we conducted a clinical follow-up by the combination of outpatient visits and telephone calls. The X-ray examination of the primary surgical site and chest was performed every 3–6 months. If necessary, computed tomography (CT) was conducted. The cut-off deadline of follow-up was October 20, 2016. Follow up lasted from 10 to 120 months with a median of 60.5 months.
Immunohistochemistry
All tissue samples were fixed in 4% formalin, decalcified in ethylene diamine tetraacetic acid (EDTA) solution, and embedded in paraffin. They were cut into 4-μm thickness sections and heated in an oven for approximately 60 min. Then, the sections were deparaffinized with xylene and rehydrated followed by a graded series of ethanol. After heat-induced antigen retrieval and endogenous peroxidase blocking, the sections were incubated with primary antibodies overnight at 4 °C. Anti-BMPR2 antibody (ab78422, monoclonal, 1:200 dilution) and anti-HIF1-α (ab16066, monoclonal, 1:400 dilution) were purchased from Abcam (Cambridge, UK). Phosphate-buffered saline (PBS) was used as a negative control. Subsequently, the slides were incubated with horseradish peroxidase-linked anti-mouse secondary antibody (ZB2305, 1:100 dilution, ZSGB-BIO, China) for 30 min at 37 °C. The universal 3,3’-diaminobenzidine detection kit (AR1026, Boster, China) was used as the final chromogen and the nucleus was counterstained by hematoxylin.
Evaluation of immunohistochemistry
According to the percentage of positively stained osteosarcoma cells, the sections were graded by four levels: 0, 0% cells; 1, <5% positive; 2, 5%–50% positive; and 3, >50% positive. Staining intensity was graded as follows: 0, none; 1, mild staining; 2, moderate staining; and 3, intense staining. Total score = percentage score × staining intensity. For both parameters, two independent pathologists blinded from clinical data analyzed the immunoreactivity. Based on the total score, scores of 0–3 was considered as negative expression, and scores of 4–9 was represented positive expression.
Statistical analysis
The SPSS software package (Version 17.0; SPSS Inc., Chicago, IL, USA) was used for all statistical analysis. Association of BMPR2 and/or HIF1-α and the clinicopathological data were analyzed by Chi-squared test. The correlation analysis for BMPR2 and HIF1-α expression was assessed by the non-parametric Spearman test. The predictive value of BMPR2 and HIF1-α expression in metastasis was evaluated by receiver-operating characteristic (ROC) curves, and the Cox model was used. Overall survival (OS) was compared among the groups with Kaplan-Meier method (log-rank test), and multivariate survival analysis was performed using the Cox proportional hazard model. Differences with P<0.05 was considered as statistically significant.
Results
Patient characteristics
The clinical and pathological characteristics are presented in Table 1. Forty-nine males and 54 females osteosarcoma patients were enrolled in our study, ranging in age from 7 to 55 (mean: 18) years. The distributions of tumor location were as follows: femur (n=39), tibia (n=23), humerus (n=23) and other locations (n=18). Histologically, the specimens were classified into osteoblastic group (n=61), chondroblastic group (n=25) and other groups (n=17). According to the pathological classification, 48 osteosarcoma patients were confirmed to be grade I, and 55 patients were grade II and III. A total of 57 osteosarcoma patients exhibited lung metastasis until the end of follow-up.
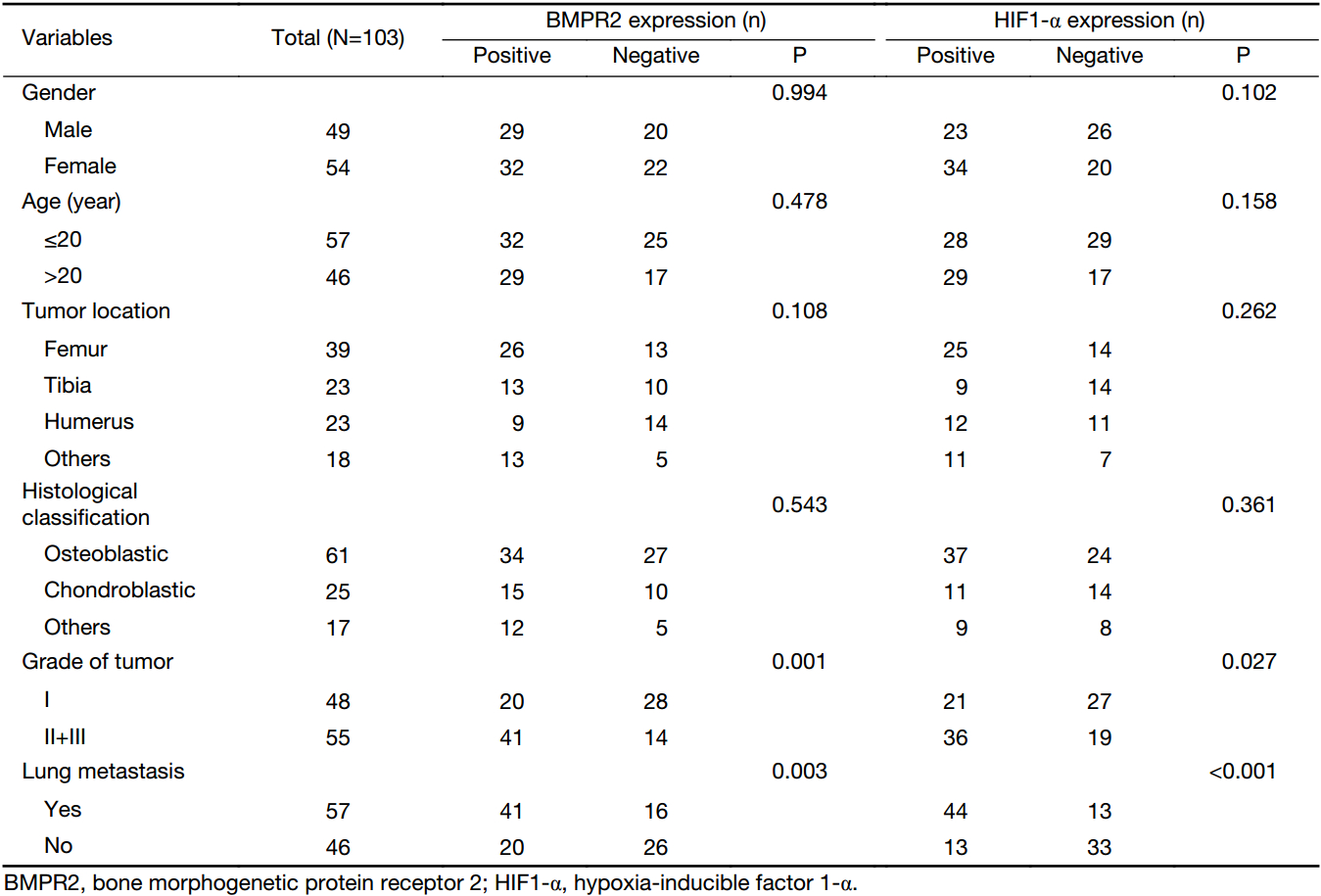
Full table
Expression of BMPR2 and HIF1-α in osteosarcoma patients
The expression of BMPR2 and HIF1-α was detected by immunohistochemistry in osteosarcoma specimens. As shown in Figure 1, the different expression levels of BMPR2 and HIF1-α in osteosarcoma samples are presented. Yellow or brown indicates positive expression of the marker. Positive staining of BMPR2 was predominantly examined in the nucleus and cytoplasm (Figure 1A), and the location of HIF1-α expression was identified in the nucleus and cytoplasm (Figure 1C). The positive rates of the BMPR2 and HIF1-α expression were 59.2% (61/103) and 55.3% (57/103), respectively. We found that positive expressions of BMPR2 and HIF1-α were significantly associated with tumor grade (P=0.001 and P=0.027, respectively) and distant metastasis (P=0.003 and P<0.001, respectively). However, the expression of BMPR2 and HIF1-α had no correlation with gender, age, tumor location and histological classification (Table 1).
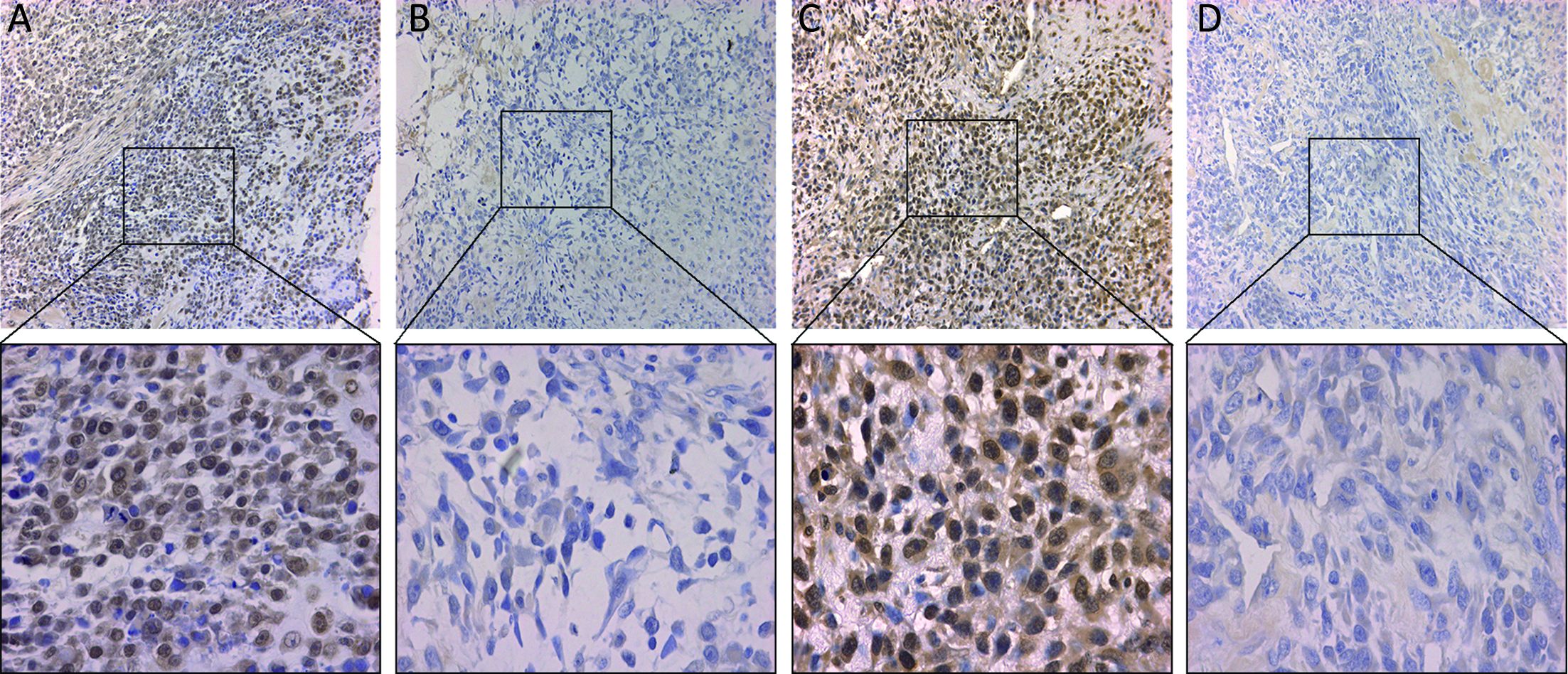
As indicated in Table 2, there were 39 (37.9%) osteosarcoma patients with BMPR2 and HIF1-α co-positive expression and 24 (23.3%) patients with BMPR2 and HIF1-α co-negative expression. In addition, there were 30 (29.1%) patients with either BMPR2- or HIF1-α-positive staining. Correlation analysis was used to examine whether BMPR2 and HIF1-α were associated with each other in osteosarcoma. This result disclosed that there was a significant correlation between BMPR2 and HIF1-α expression (P=0.035, Table 2).
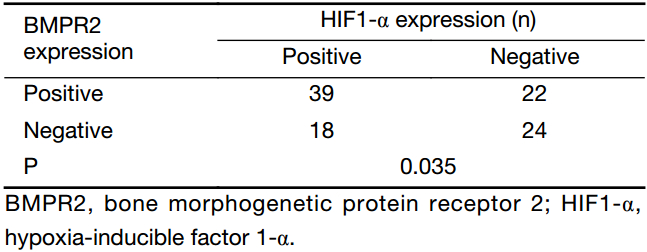
Full table
Predictive value of BMPR2 and HIF1-α in osteosarcoma metastasis
Our study found that the positive rate of BMPR2 and HIF1-α expression in osteosarcoma samples with lung metastasis was higher than that of non-metastatic specimens. Therefore, we hypothesized that BMPR2 and HIF1-α might be biomarkers for predicting pulmonary metastasis. ROC curves were used to evaluate this. We found that the area under the ROC curve for BMPR2 and HIF1-α was 0.682 and 0.790, respectively (Table 3). In addition, the area of the combined group was 0.854, indicating that the combined detection of BMPR2 and HIF1-α was more valuable in predicting lung metastasis.
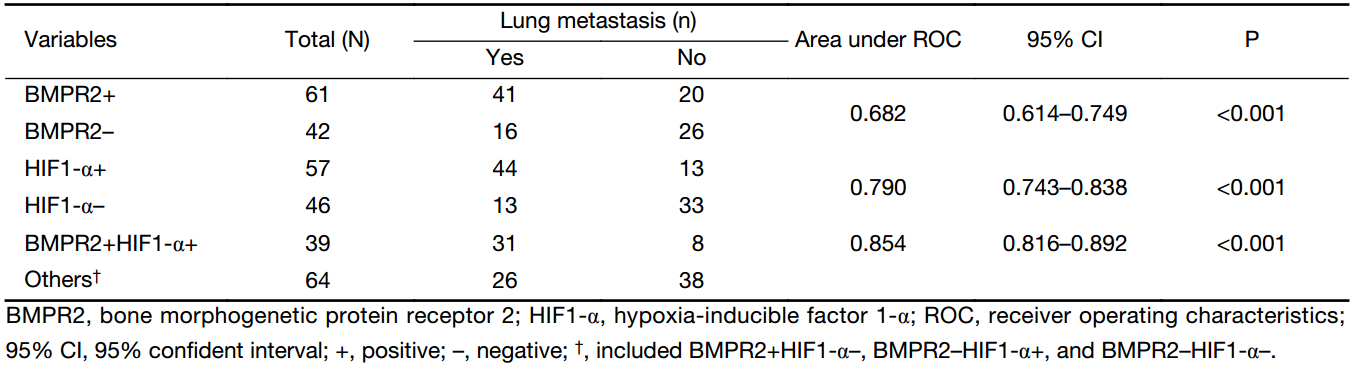
Full table
Relationship between BMPR2 and HIF1-α expression and patients’ OS
The OS in our study was 48.5% (50/103) until the deadline for follow-up. Patients with BMPR2-positive tumors had a significantly lower OS than those with BMPR2-negative tumors (44.3% and 54.8%, respectively, P=0.001) (Figure 2A). Similarly, the OS in the HIF1-α positive group was significantly lower than that in the HIF1α-negative group (33.3% and 67.4%, respectively, P<0.001) (Figure 2B). In addition, survival analysis revealed that the co-positive expression of the BMPR2 and HIF1-α group had the lowest survival compared with the other two groups (P<0.001) (Figure 2C).
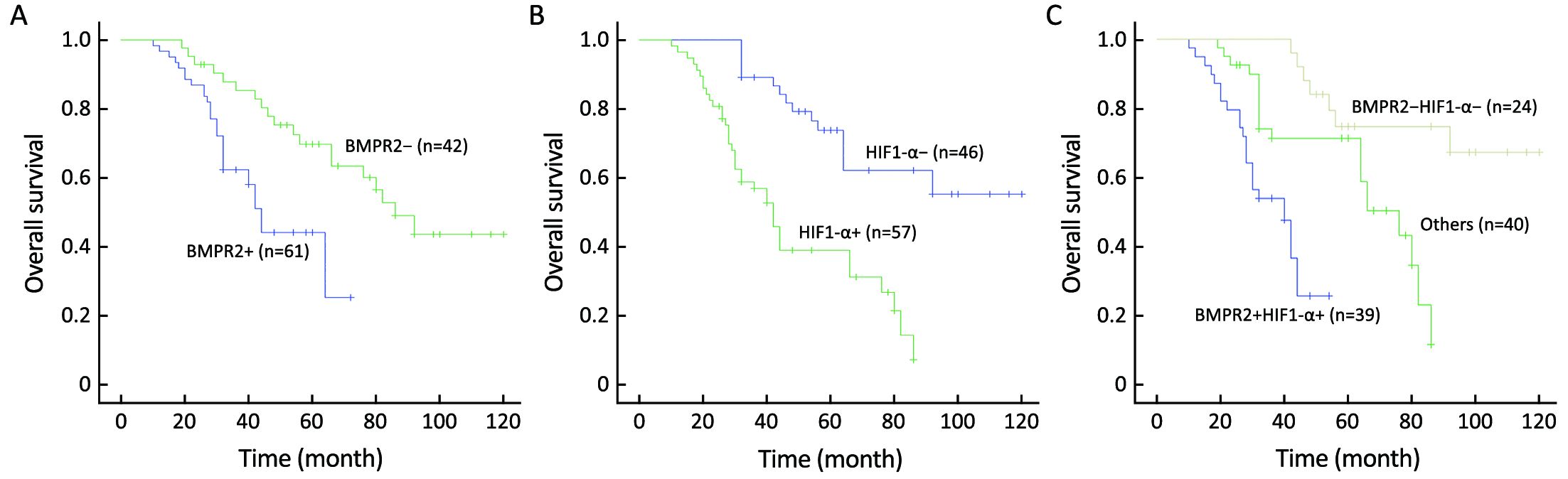
Furthermore, univariate and multivariate analyses were conducted to observe the factors related to the prognosis of osteosarcoma patients. Our data revealed that BMPR2-positive expression, HIF1-α positive expression, and higher tumor grade were correlated with the OS of osteosarcoma patients (P=0.046, P<0.001, P=0.007, respectively) (Table 4). However, there was no correlation between OS and age, gender, tumor location and histological classification (Table 4).
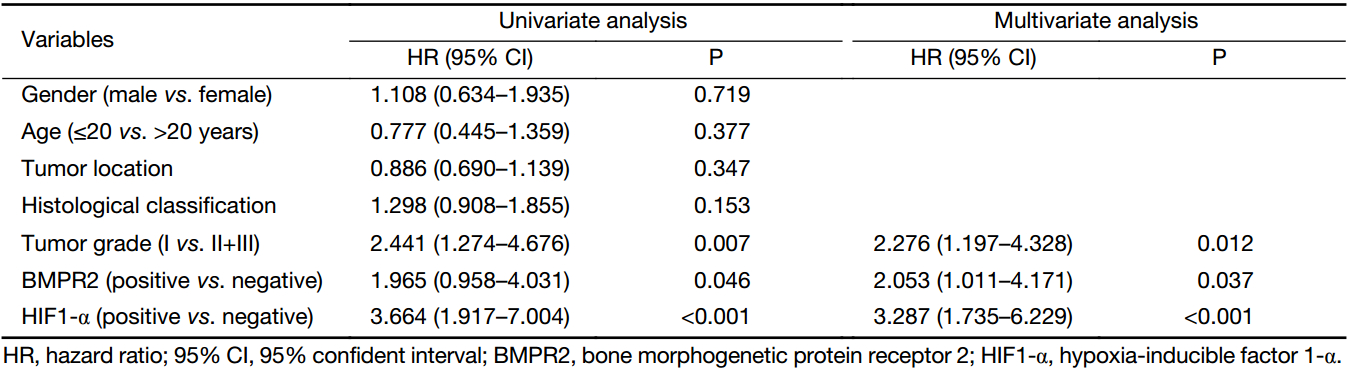
Full table
Discussion
Osteosarcoma is a locally aggressive malignancy characterized by rapid high rates of lung metastasis. The excessive growth of malignant tumors leads to a hypoxic microenvironment for tumor cells, which has an obvious influence on the biological behavior and prognosis of tumors. Previous studies revealed that intratumoral hypoxia not only cripples the clinical effect of radiotherapy and chemotherapy but also increases the risk of invasion and distant metastasis (18).
HIF1, a heterodimeric nuclear transcription factor, is widely distributed in mammals and humans under hypoxic conditions. HIF1 is composed of α and β subunits, of which α is the only regulatory subunit, which determines the activity of HIF1 and activates the expression of various hypoxia response genes and is regulated and influenced by various factors (19). It has been demonstrated that HIF1-α overexpression was detected in a variety of solid tumors (20). Meanwhile, recent studies have shown that in oral squamous cell carcinoma (21), esophageal squamous cell carcinoma (22), renal clear cell carcinoma (23) and breast cancer (24), HIF1-α expression was correlated with cancer metastasis. In our study, we demonstrated that 57 cases of 103 samples were HIF1-α-positive, and consistent with previous findings (25), HIF1-α levels were associated with lung metastasis in osteosarcoma patients (P<0.001).
It is well known that HIF1-α expression is closely related to TGF-β/BMP signaling pathways. For example, HIF1-α mediates TGF-β1-induced Snail and TWIST1 expression in breast cancer cells (26). Qian et al. (27) reported that TGF-β1 affects the nuclear translocation activity of HIF1-α in NiONPs-induced pulmonary fibrosis. Similarly, mutant BMPR2 expression resulted in an increase of HIF1-α in mouse pulmonary vascular endothelial cells (28). Whether this correlation exists in osteosarcoma is unknown; therefore, we performed this research.
As disclosed above, BMPR2 and HIF1-α were overexpressed in osteosarcoma and there is an obvious relationship between BMPR2 and HIF1-α in osteosarcoma (P=0.035). Furthermore, the protein levels of BMPR2 in osteosarcoma were in accordance with the mRNA levels reported previously (29). In addition, BMPR2 and HIF1-α were correlated with the tumor grade. However, there was no correlation with gender, age, tumor location and histological classification. Here, it should be noted that we used a pathology classification method in our study, rather than clinical grading system, but the method of clinical classification system still needed our further analysis.
We also investigated the predictive value of BMPR2 and HIF1-α in the distant metastasis and prognosis of osteosarcoma patients. The data of ROC analysis revealed that the combined detection of BMPR2 and HIF1-α was more valuable in predicting lung metastasis. Additionally, the patients with BMPR2 and HIF1-α co-positivity had poor OS compared with other groups. These results suggested that the combined detection of BMPR2 and HIF1-α could be used as prognostic factors in osteosarcoma.
Conclusions
In general, individualized therapy targeting BMPR2 and HIF1-α together may serve as a promising modality to prevent distant metastasis and tumor progression in osteosarcoma patients. However, the underlying mechanism of BMPR2 and HIF1-α in lung metastasis and osteosarcoma invasion still calls for further investigation.
Acknowledgements
The study was supported by grants from the National Natural Science Foundation of China (No. 81572633). The funders had no role in the study design, data collection and analysis, decision to publish, or manuscript preparation.
Footnote
Conflicts of Interest: The authors have no conflicts of interest to declare.
References
- Kansara M, Teng MW, Smyth MJ, et al. Translational biology of osteosarcoma. Nat Rev Cancer 2014;14:722–35. [PubMed] DOI:10.1038/nrc3838
- Bacci G, Rocca M, Salone M, et al. High grade osteosarcoma of the extremities with lung metastases at presentation: treatment with neoadjuvant chemotherapy and simultaneous resection of primary and metastatic lesions. J Surg Oncol 2008;98:415–20. [PubMed] DOI:10.1002/jso.21140
- Wu PK, Chen WM, Chen CF, et al. Primary osteogenic sarcoma with pulmonary metastasis: clinical results and prognostic factors in 91 patients. Jpn J Clin Oncol 2009;39:514–22. [PubMed] DOI:10.1093/jjco/hyp057
- Kempf-Bielack B, Bielack SS, Jürgens H, et al. Osteosarcoma relapse after combined modality therapy: an analysis of unselected patients in the Cooperative Osteosarcoma Study Group (COSS). J Clin Oncol 2005;23:559–68. [PubMed] DOI:10.1200/JCO.2005.04.063
- Bami M, Mavrogenis AF, Angelini A, et al. Bone morphogenetic protein signaling in musculoskeletal cancer. J Cancer Res Clin Oncol 2016;142:2061–72. [PubMed] DOI:10.1007/s00432-016-2149-9
- Sieber C, Kopf J, Hiepen C, et al. Recent advances in BMP receptor signaling. Cytokine Growth Factor Rev 2009;20:343–55. [PubMed] DOI:10.1016/j.cytogfr.2009.10.007
- Kim IY, Lee DH, Lee DK, et al. Loss of expression of bone morphogenetic protein receptor type II in human prostate cancer cells. Oncogene 2004;23:7651–9. [PubMed] DOI:10.1038/sj.onc.1207924
- Beppu H, Mwizerwa ON, Beppu Y, et al. Stromal inactivation of BMPRII leads to colorectal epithelial overgrowth and polyp formation. Oncogene 2008;27:1063–70. [PubMed] DOI:10.1038/sj.onc.1210720
- Pouliot F, Blais A, Labrie C. Overexpression of a dominant negative type II bone morphogenetic protein receptor inhibits the growth of human breast cancer cells. Cancer Res 2003;63:277–81. [PubMed]
- Jiao G, Guo W, Ren T, et al. BMPR2 inhibition induced apoptosis and autophagy via destabilization of XIAP in human chondrosarcoma cells. Cell Death Dis 2014;5:e1571. [PubMed] DOI:10.1038/cddis.2014.540
- El-Naggar AM, Veinotte CJ, Cheng H, et al. Translational activation of HIF1α by YB-1 promotes sarcoma metastasis. Cancer Cell 2015;27:682–97. [PubMed] DOI:10.1016/j.ccell.2015.04.003
- Finger EC, Giaccia AJ. Hypoxia, inflammation, and the tumor microenvironment in metastatic disease. Cancer Metastasis Rev 2010;29:285–93. [PubMed] DOI:10.1007/s10555-010-9224-5
- Wilson WR, Hay MP. Targeting hypoxia in cancer therapy. Nat Rev Cancer 2011;11:393–410. [PubMed] DOI:10.1038/nrc3064
- Semenza GL. Hypoxia-inducible factor 1 and cancer pathogenesis. IUBMB Life 2008;60:591–7. [PubMed] DOI:10.1002/iub.93
- Masoud GN, Li W. HIF-1α pathway: role, regulation and intervention for cancer therapy. Acta Pharm Sin B 2015;5:378–89. [PubMed] DOI:10.1016/j.apsb.2015.05.007
- Keith B, Johnson RS, Simon MC. HIF1α and HIF2α: sibling rivalry in hypoxic tumour growth and progression. Nat Rev Cancer 2011;12:9–22. [PubMed] DOI:10.1038/nrc3183
- Guo M, Cai C, Zhao G, et al. Hypoxia promotes migration and induces CXCR4 expression via HIF-1α activation in human osteosarcoma. PLoS One 2014;9:e90518. [PubMed] DOI:10.1371/journal.pone.0090518
- Hutchison GJ, Valentine HR, Loncaster JA, et al. Hypoxia-inducible factor 1α expression as an intrinsic marker of hypoxia: correlation with tumor oxygen, pimonidazole measurements, and outcome in locally advanced carcinoma of the cervix. Clin Cancer Res 2004;10:8405–12. [PubMed] DOI:10.1158/1078-0432.CCR-03-0135
- Nepal M, Choi HJ, Choi BY, et al. Anti-angiogenic and anti-tumor activity of Bavachinin by targeting hypoxia-inducible factor-1α. Eur J Pharmacol 2012;691:28–37. [PubMed] DOI:10.1016/j.ejphar.2012.06.028
- Zhong H, De Marzo AM, Laughner E, et al. Overexpression of hypoxia-inducible factor 1α in common human cancers and their metastases. Cancer Res 1999;59:5830–5. [PubMed]
- Ishikawa T, Nakashiro K, Klosek SK, et al. Hypoxia enhances CXCR4 expression by activating HIF-1 in oral squamous cell carcinoma. Oncol Rep 2009;21:707–12. [PubMed]
- Kurokawa T, Miyamoto M, Kato K, et al. Overexpression of hypoxia-inducible-factor 1α (HIF-1α) in oesophageal squamous cell carcinoma correlates with lymph node metastasis and pathologic stage. Br J Cancer 2003;89:1042–7. [PubMed] DOI:10.1038/sj.bjc.6601186
- Wan L, Huang J, Chen J, et al. Expression and significance of FOXP1, HIF-1a and VEGF in renal clear cell carcinoma. J BUON 2015;20:188–95. [PubMed]
- Cai FF, Xu C, Pan X, et al. Prognostic value of plasma levels of HIF-1a and PGC-1a in breast cancer. Oncotarget 2016;7:77793–806. [PubMed] DOI:10.18632/oncotarget.12796
- Yang QC, Zeng BF, Dong Y, et al. Overexpression of hypoxia-inducible factor-1α in human osteosarcoma: correlation with clinicopathological parameters and survival outcome. Jpn J Clin Oncol 2007;37:127–34. [PubMed] DOI:10.1093/jjco/hyl137
- Cho KH, Yu SL, Cho DY, et al. Breast cancer metastasis suppressor 1 (BRMS1) attenuates TGF-β1-induced breast cancer cell aggressiveness through downregulating HIF-1α expression. BMC Cancer 2015;15:829. [PubMed] DOI:10.1186/s12885-015-1864-y
- Qian F, He M, Duan W, et al. Cross regulation between hypoxia-inducible transcription factor-1α (HIF-1α) and transforming growth factor (TGF)-β1 mediates nickel oxide nanoparticles (NiONPs)-induced pulmonary fibrosis. Am J Transl Res 2015;7:2364–78. [PubMed]
- Bryant AJ, Robinson LJ, Moore CS, et al. Expression of mutant bone morphogenetic protein receptor II worsens pulmonary hypertension secondary to pulmonary fibrosis. Pulm Circ 2015;5:681–90. [PubMed] DOI:10.1086/683811
- Guo W, Gorlick R, Ladanyi M, et al. Expression of bone morphogenetic proteins and receptors in sarcomas. Clin Orthop Relat Res 1999:175–83. [PubMed]